Project Category: Chemical
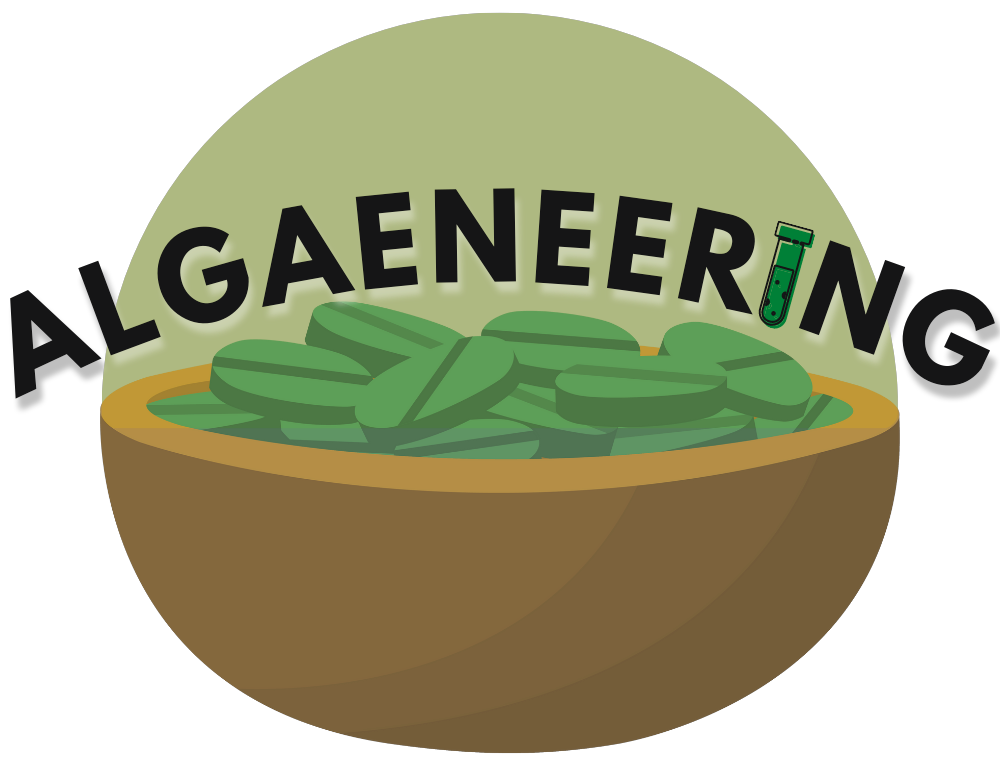

View Our Presentation
April 5, 9:30 AM to 1:30 PM
Atrium (G block) at the Schulich School of Engineering
Learn About Our Project
ABOUT OUR PROJECT
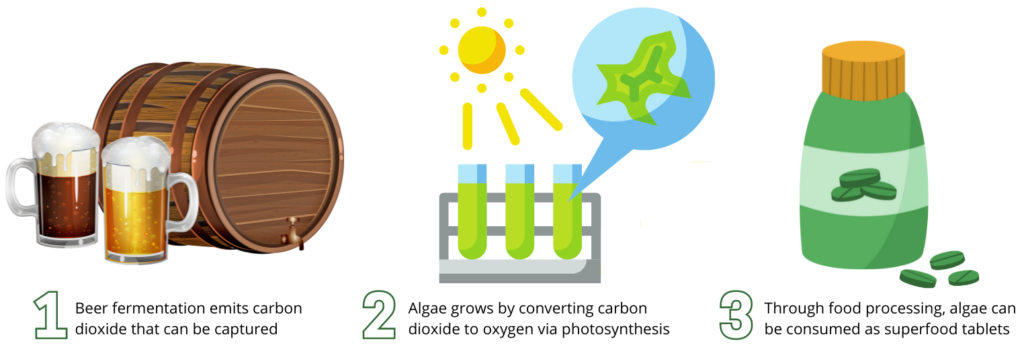
CARBON DIOXIDE capture FROM BEER FERMENTATION
The fermentation of yeast with barley hops, and water, produces a delicious drink, but the process also produces carbon dioxide as a by-product. Carbon dioxide is one of the major contributors to green house gas emissions. Fahr plans to capture 90% of the carbon dioxide produced during their beer fermentation process and significantly reduce their emissions.
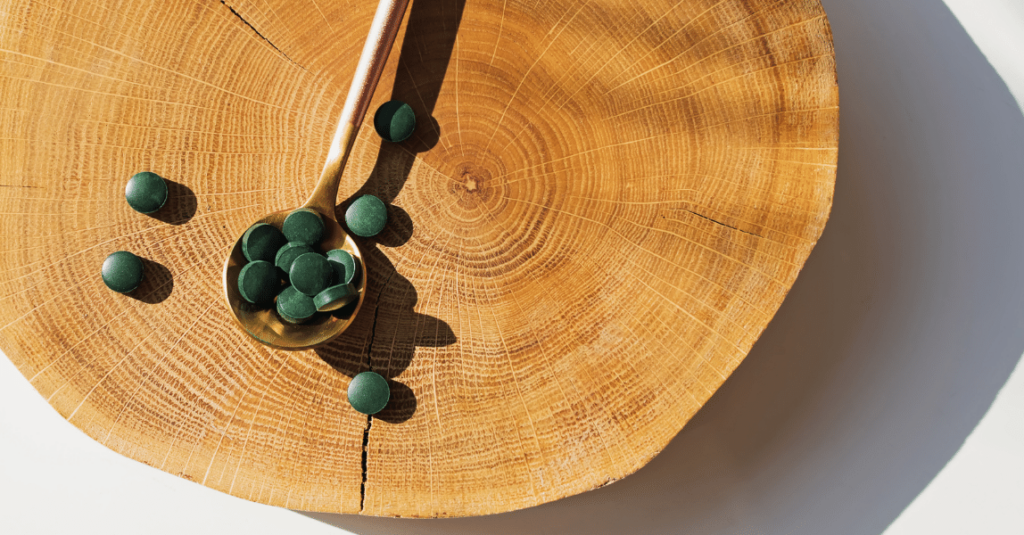
Other Benefits
Another benefit to Fahr producing algae from beer fermentation carbon dioxide is that algae turns carbon dioxide into oxygen when undergoing photosynthesis. The beer brewing process requires carbon dioxide and oxygen at different stages in controlled amounts, so currently Fahr is purchasing both externally but with Algaeneering’s process, Fahr can produce them in-house.

Growing Chlorella Vulgaris Algae
Chlorella vulgaris is an algae than can grow at room temperature at atmospheric pressures in the presence of carbon dioxide and certain nutrients. It can grow in any water source, but for quality control Fahr will be growing it in photobioreactors. The Chlorella vulgaris can be processed into a green powder for consumption as a superfood, but many say the green powder is gross! Fahr will be drying, crushing, and pressing the algae into a pill so consumers can enjoy the benefits without the taste.

Details about our design
DESIGN OVERVIEW
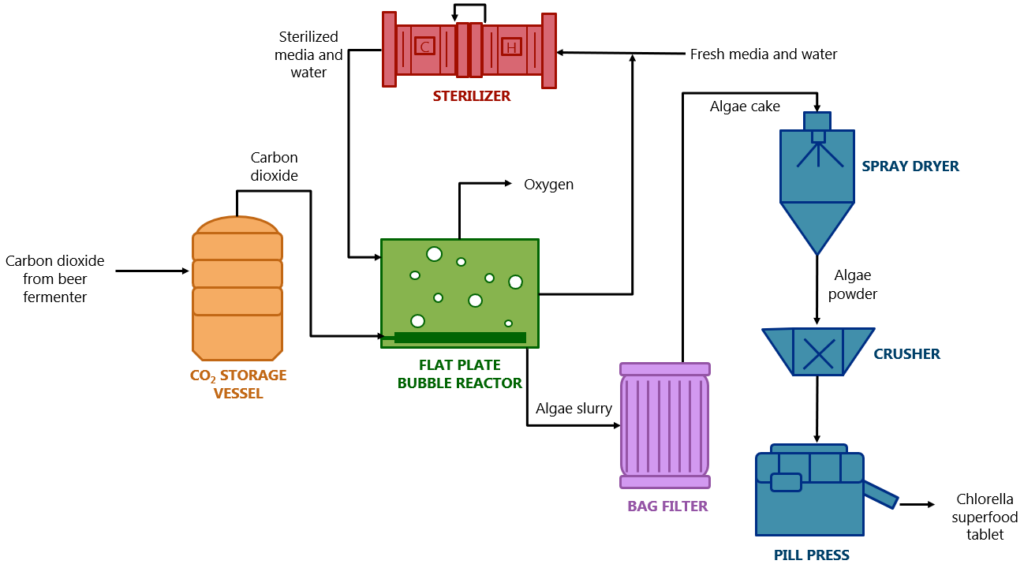
1. CO2 from the beer fermenter is captured, filtered to remove yeast and contaminants, and is stored until needed
2. The sterilizer quickly heats up the water and medium to kill off any bacteria in the water then cools down the mixture to be fed to the reactor
3. The transparent flat plate reactor takes water and medium as well as light and by bubbling CO2, undergoes a photosynthesis reaction to grow Chlorella vulgaris algae
4. The algae slurry exits the reactor and goes through a bag filter where excess water is removed to give an algae cake
5. The algae cake is dried by a spray dryer to produce an algae powder that can then be crushed and pressed into algae tablets
HOW OUR DESIGN ADDRESSES PRACTICAL ISSUES
This design takes a byproduct from a beer fermentation facility (CO2) and sequesters it by using it to grow algae that can be sold as a superfood. With the government of Canada aiming for net-zero greenhouse gas (GHG) emissions by 2050, this process will help with the long road to address this issue by decreasing harmful emissions. Additionally, the Alberta carbon tax will increase by $10/ton each year until reaching $170/ton in 2030 (Canada Drives, 2021). Breweries can save money by not having to pay these increasing carbon taxes by decreasing their CO2 byproduct, which reduces this expense for Fahr brewery while creating a new revenue stream. Therefore, this process addresses our client’s issue of having to pay carbon tax on the emissions released from their facility and the environmental impact of releasing CO2 emissions into the atmosphere while increasing overall profits. Not only can breweries save money by eliminating their CO2, but they can also potentially be granted funding to help pay for the implementation of their carbon reduction systems. Currently, over $500 million is available through the Low Carbon Economy Challenge, to support projects aimed to reduce emissions and generate clean growth across the country (Government of Canada, 2018).
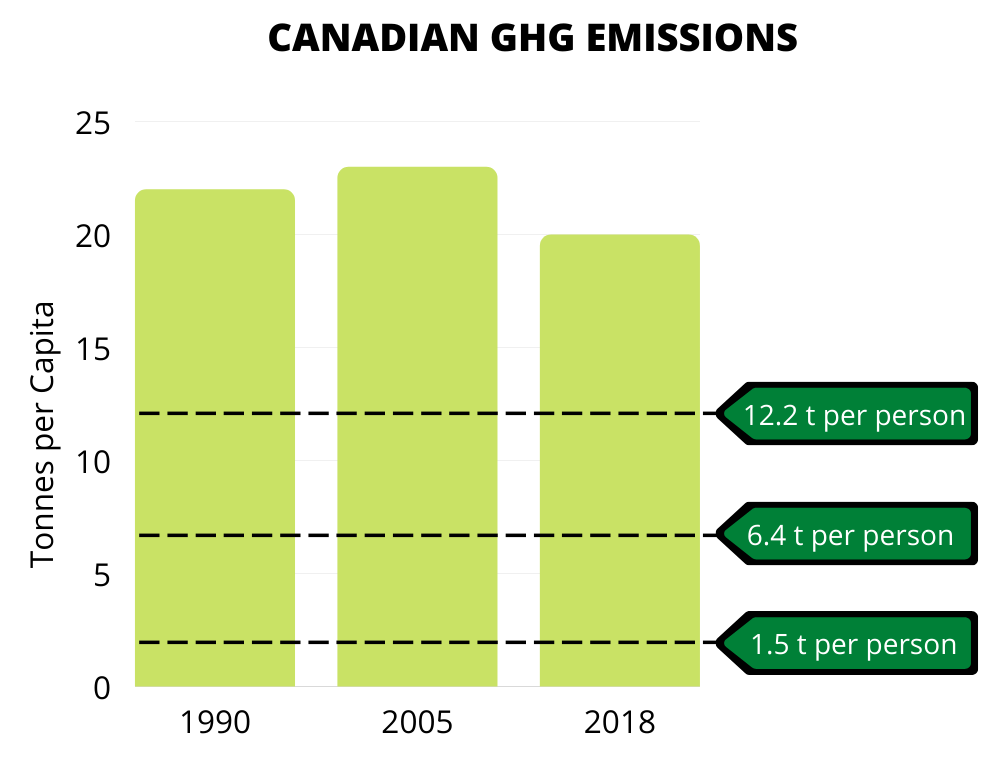
The right-side markers indicate a 30% reduction from 2005 based on 2030 population (12.2t per person), a 60% reduction from 2005 based on 2040 population (6.4t per person), a 90% reduction from 2005 based on 2050 population (1.5t per person).
WHAT MAKES OUR DESIGN INNOVATIVE
Typically captured carbon is stored in tanks or underground until required for use. However, Algaeneering’s brewery carbon capture design uses carbon dioxide to grow algae which is then processed into superfood tablets. Thus, unlike conventional carbon capture designs, Algaeneering is able to create a useful product to bring in extra profits for the Fahr brewery while providing a high-in-demand superfood for consumers.
Alberta produces more carbon than any other province in Canada so it is critical that the province finds ways to offset emissions. Alberta, along with many other Canadian provinces must abide by the federal carbon tax, which applies to all emitters that do not meet the federal standards. In the coming years, the carbon tax rate will rise will increase exponentially so Fahr beer can save money by eliminating their carbon dioxide byproduct and thus, the need to pay carbon taxes.
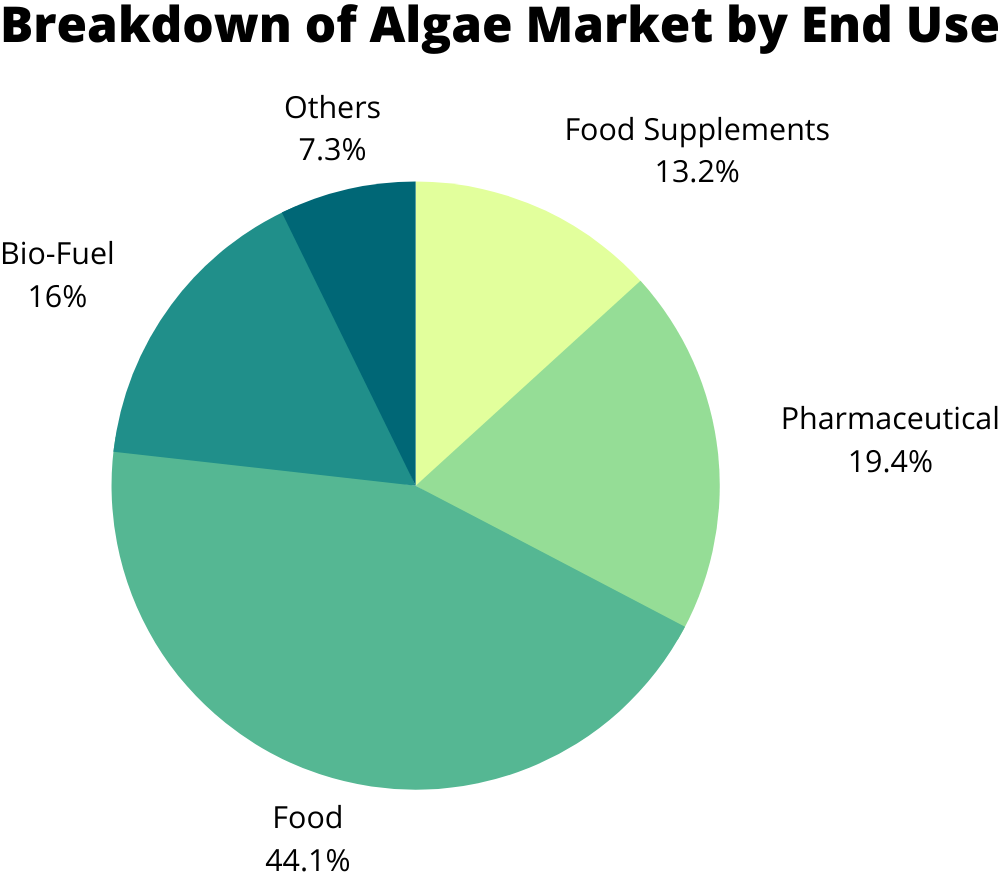
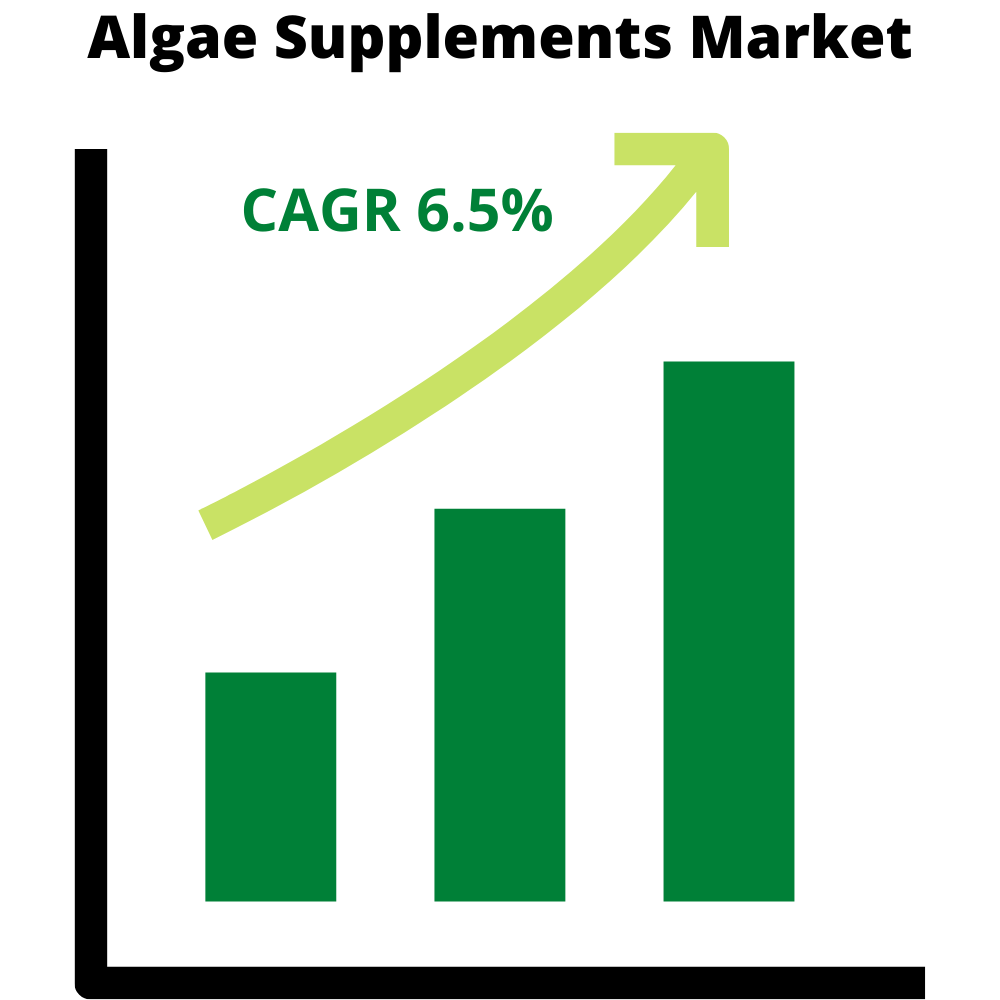
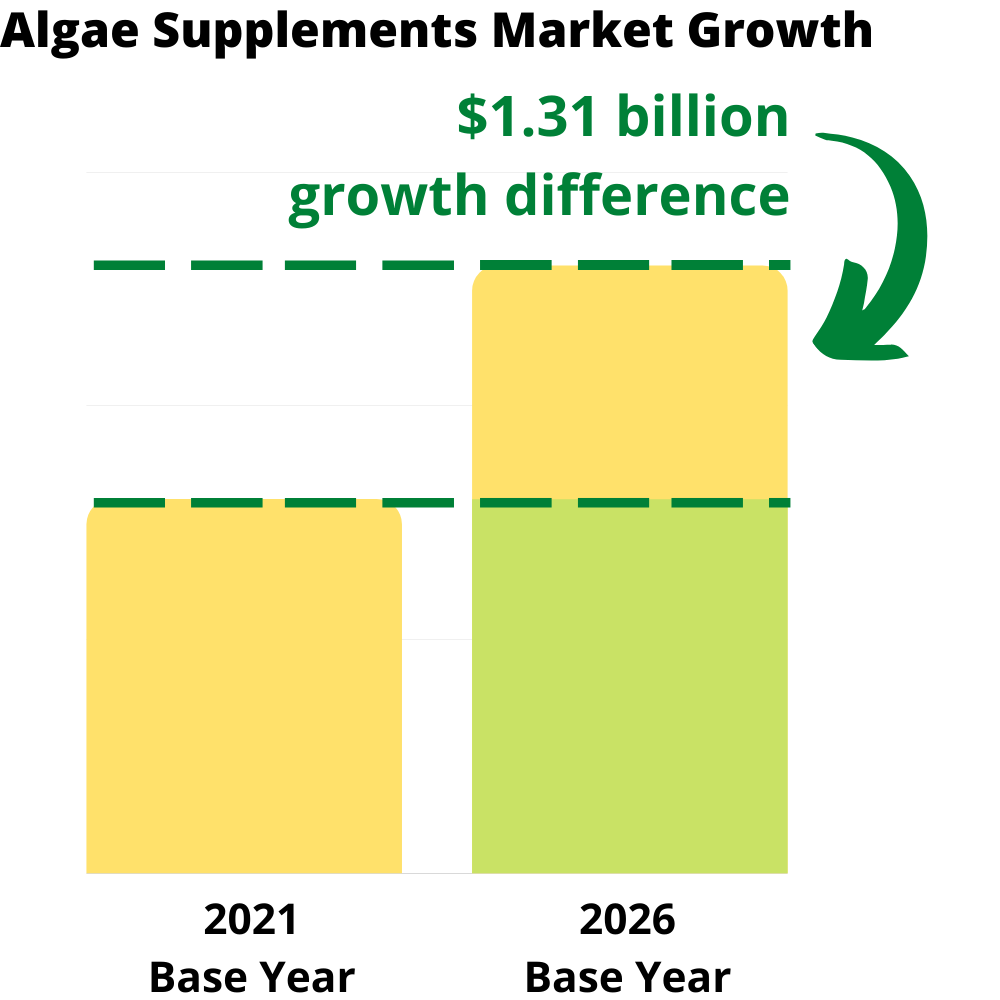
Tablet products are expected to have the fastest annual growth rate of 7.9% from 2021-2026 (IndustryARC, 2020). In addition, during the COVID-19 pandemic, consumers were searching for immune-boosting health products to lower their chance of contracting the virus, thus, amplifying the growth of the market in 2020. Next, the beauty industry uses algae proteins and supplements for their anti-aging effects and better maintenance of gut bacteria which can have beneficial results for dermal health and appearance. Lastly, changing lifestyle trends such as the increase of plant-based diets has shifted the preference for animal protein sources to plant protein sources, making chlorella tablets a highly sought after product.
WHAT MAKES OUR DESIGN SOLUTION EFFECTIVE
The beer fermentation process is a biogenic process. It involves using barley and yeast to produce beer, but the yeast also produces large amounts of CO2 as a by-product. Barley consumes CO2 to grow, so many might say that beer fermentation is already a net zero process. With CO2 being released back into the atmosphere breweries have an opportunity to capture and eliminate the CO2 produced in the fermentation process, while continuing to eliminate CO2 by growing the barley.
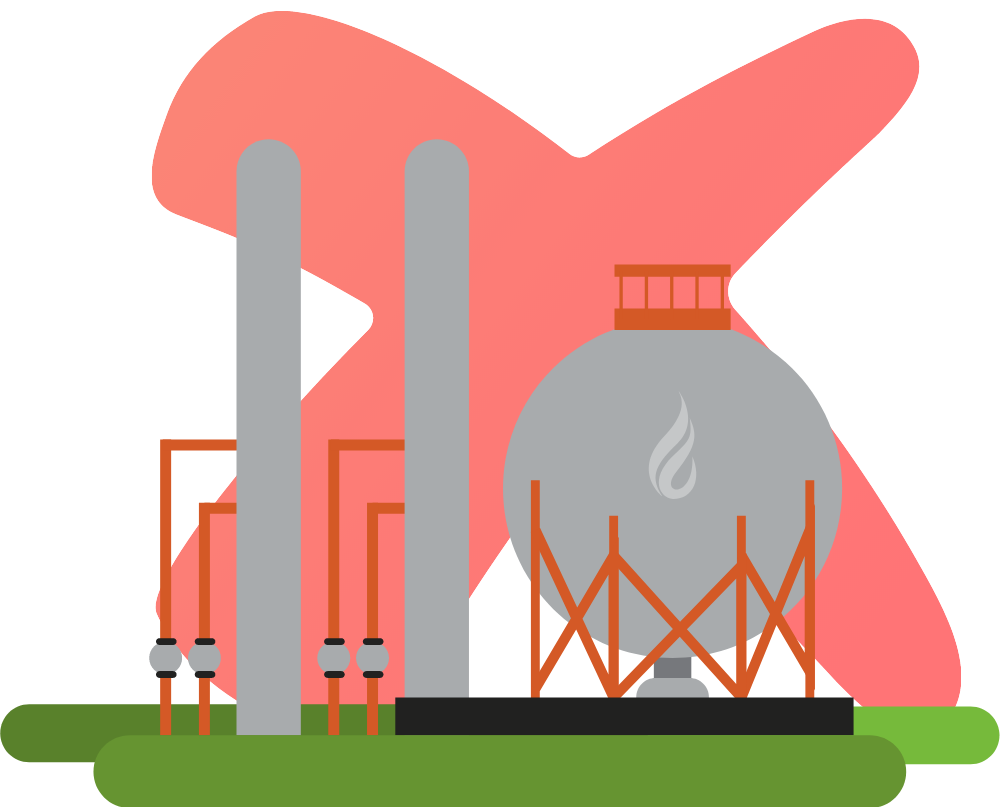
The intent of the implementation of this process is to reduce CO2 emissions. In order to optimize the amount of CO2 that is eliminated from the atmosphere we have designed a process that emits as little CO2 as possible. This process contains equipment such as a heat exchanger, conveyors, and pumps that all require energy sources to operate. If we use electricity generated from natural gas, our process would not be as environmentally friendly as one may think, because electricity from natural gas releases a significant amount of CO2 when the natural gas is burnt.
In order to ensure our process does not require electricity from a natural gas source we confirmed with our client that the electricity to the site, and that will be feeding our process, comes from wind power energy sources. In the future our client wants to produce their own electricity via solar panels, which is also an environmentally friendly source of electricity that does not release unnecessary CO2 into the atmosphere.
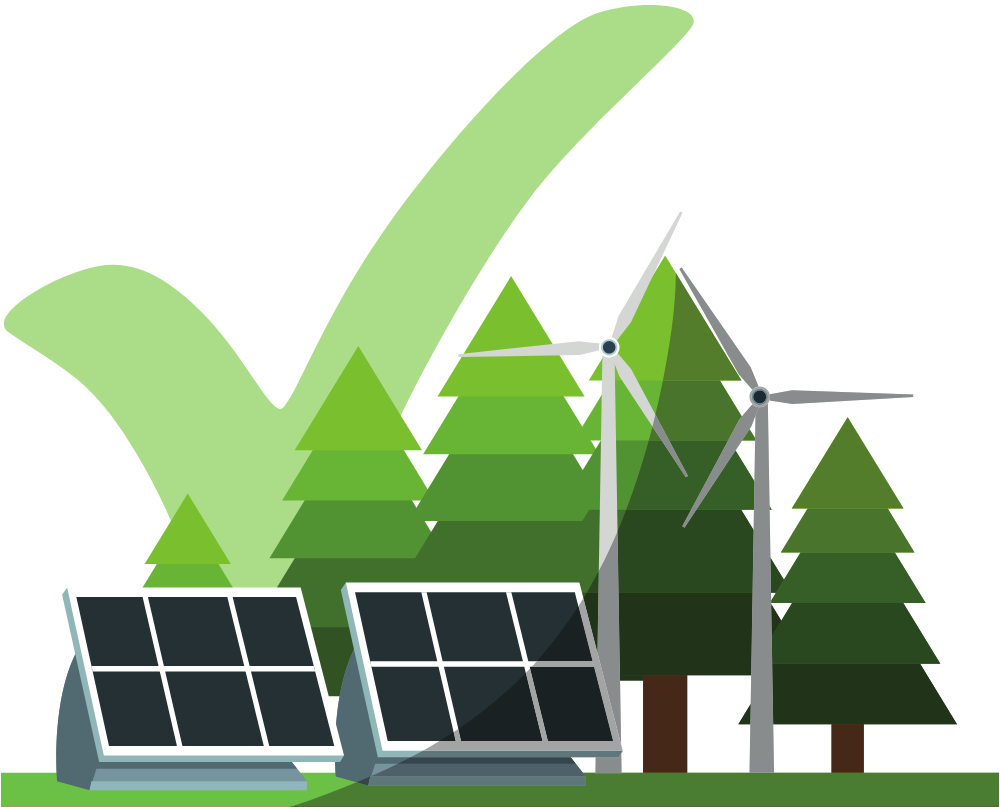
HOW WE VALIDATED OUR DESIGN SOLUTION
Reactor Simulation
In the reactor kinetic simulations that were performed we found that if we decrease the total volume of fluid in our reactors, the temperature inside the reactors gets too high to support algal growth. We determined that a larger total fluid volume is necessary to avoid needing cooling jackets. We also found that culturing algae at a higher concentration allows us to require less reactors in our process (conserving space) and improves algal growth. Finally, we found that Chlorella vulgaris grows better with less dissolved CO2.
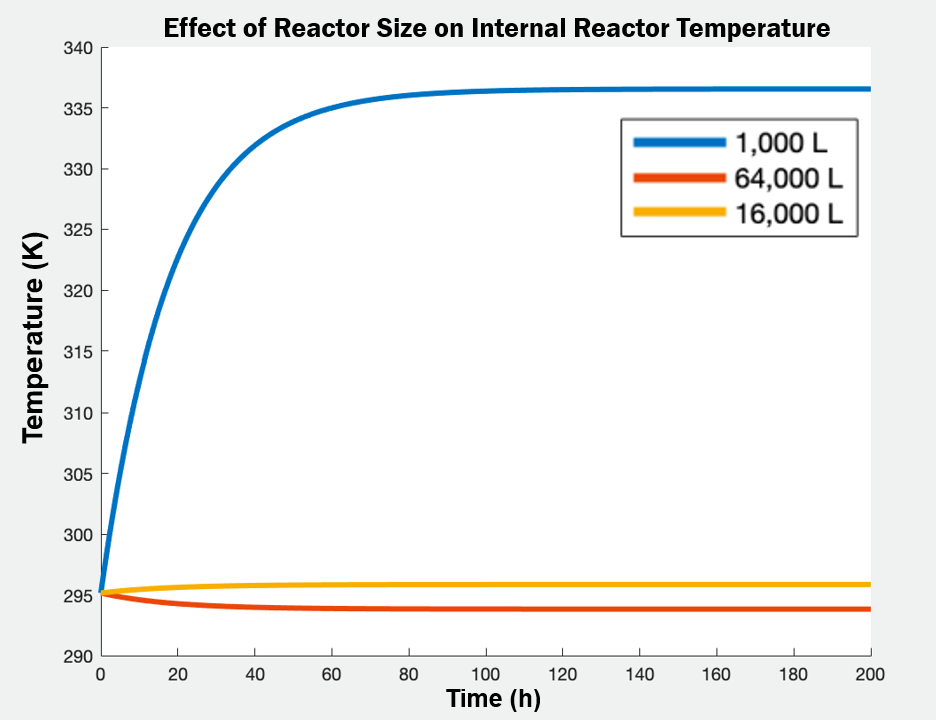
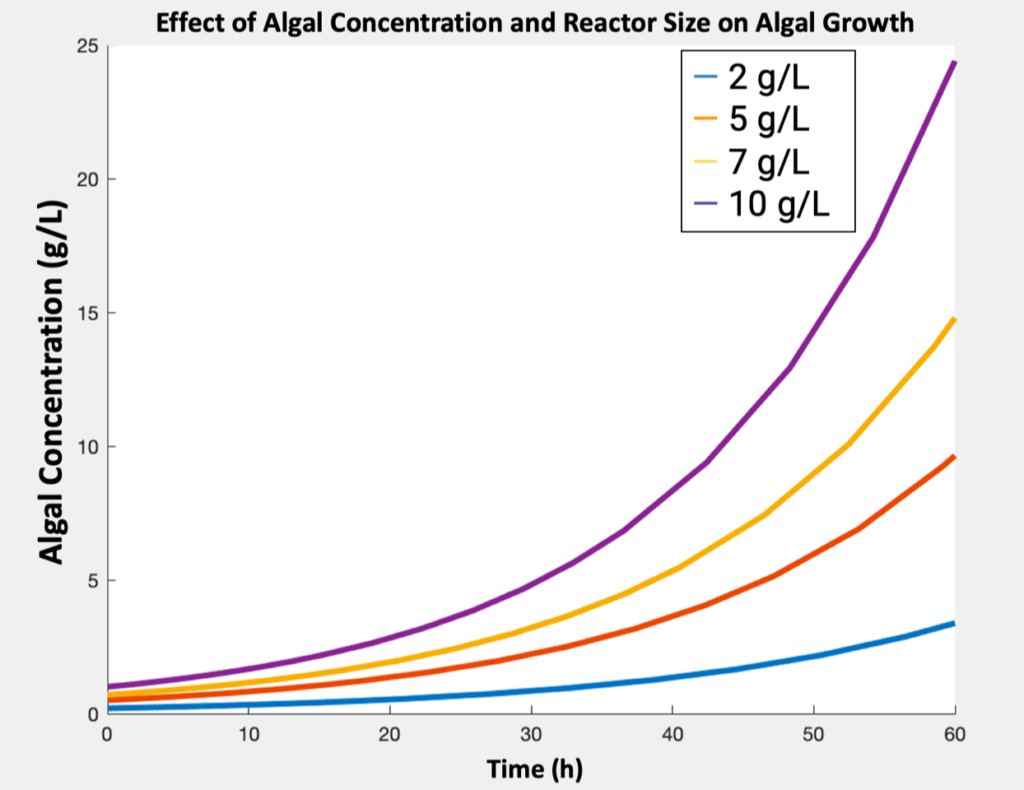
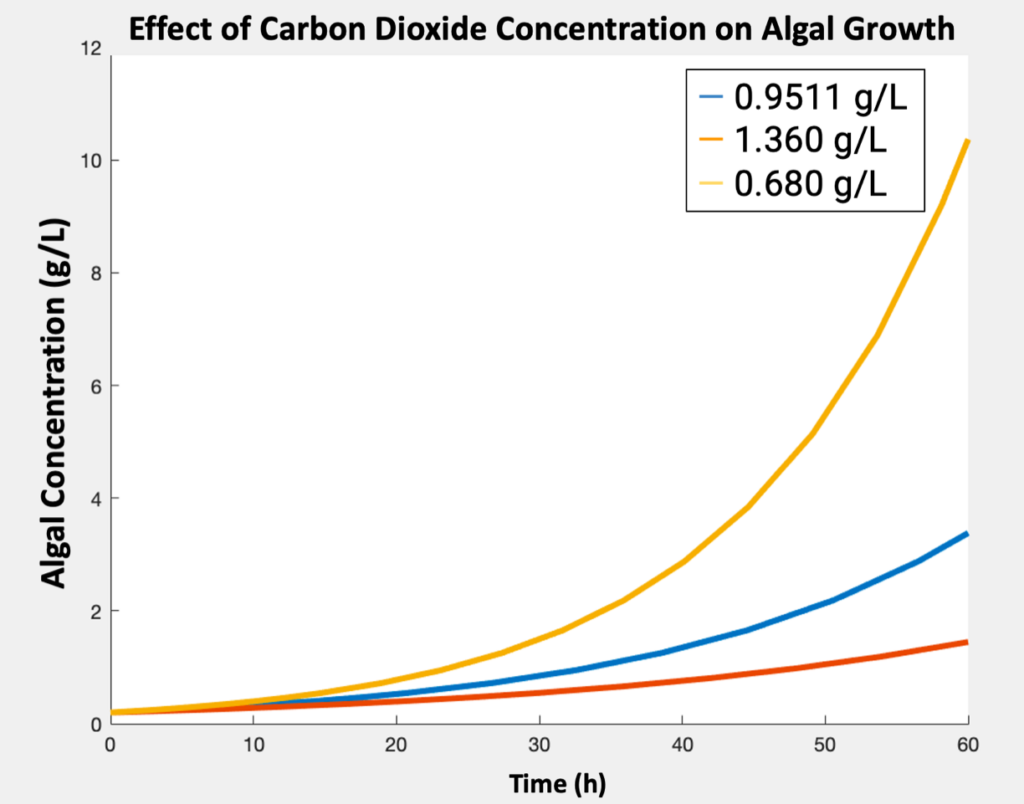
Prototype
We have built a reactor prototype for our client to test and confirm all the values that have been determined, such as light intensity and photoperiods, cultivation amounts and periods and any other information that may be useful for our design. The dimensions of our prototype are 0.5m (L) by 0.03m (W) by 1m (H). The width was calculated with the Beer Lambert equation and the height was calculated using the Navier Stokes equation. The length is not dependent on anything other than the size of the facility that the reactor will be in, so an arbitrary value was chosen for the prototype. Acrylic glue was used to seal the acrylic walls together and 4 support pieces were placed in the center to keep the structure from caving inwards.
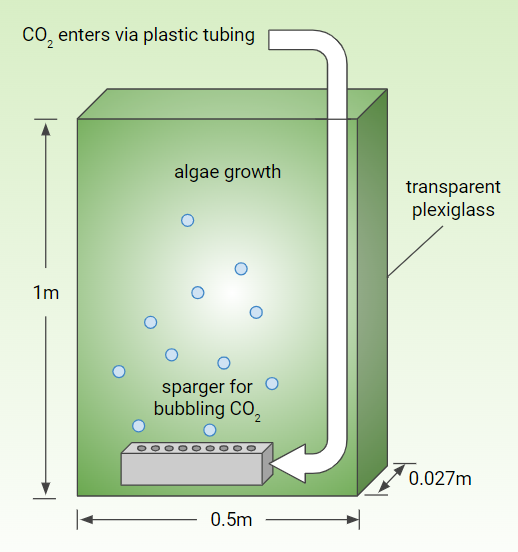
FEASIBILITY OF OUR DESIGN SOLUTION
Our design has gone through many iterations in order to make it feasible. We came to the conclusion that we will be capturing 90% of the CO2 emissions produced by the beer fermentation process of our client. Although the goal was to capture as close to 100% of the CO2 emissions as possible, the feasibility of our design decreased exponentially if we were to exceed 90%. By graphing the rate of reaction against the conversion of CO2, it was observed that the graph starts to increase exponentially at the 90% point, meaning that the growth rate of Chlorella vulgaris increases significantly at this point, which would require far too many reactors in our process. In order to maintain a feasible volume for our process, we made the decision to capture 90% of the CO2 produced by our client, giving us a volume of approximately 15,500L, with 48 reactors containing 324L each.
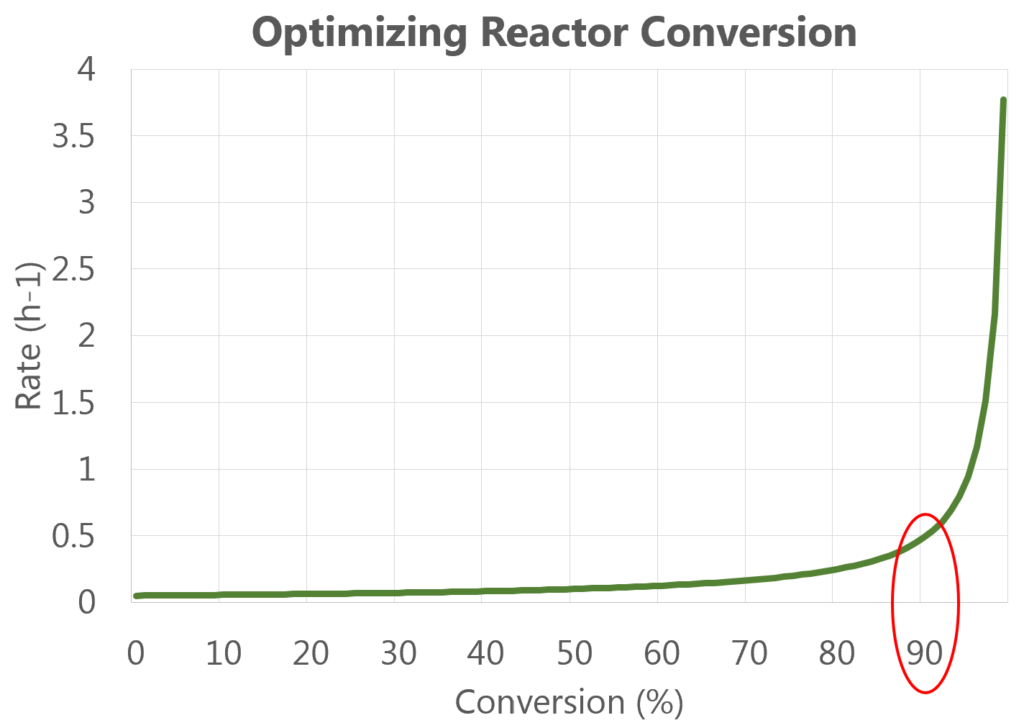
ECONOMICS OF OUR DESIGN
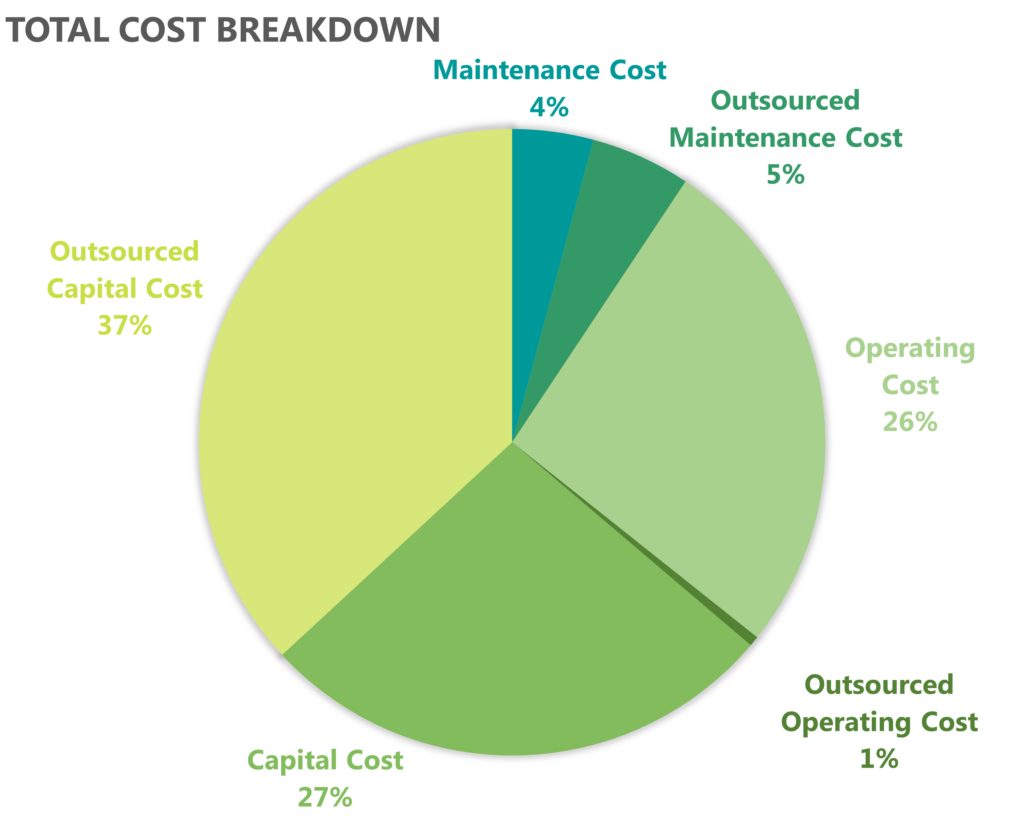
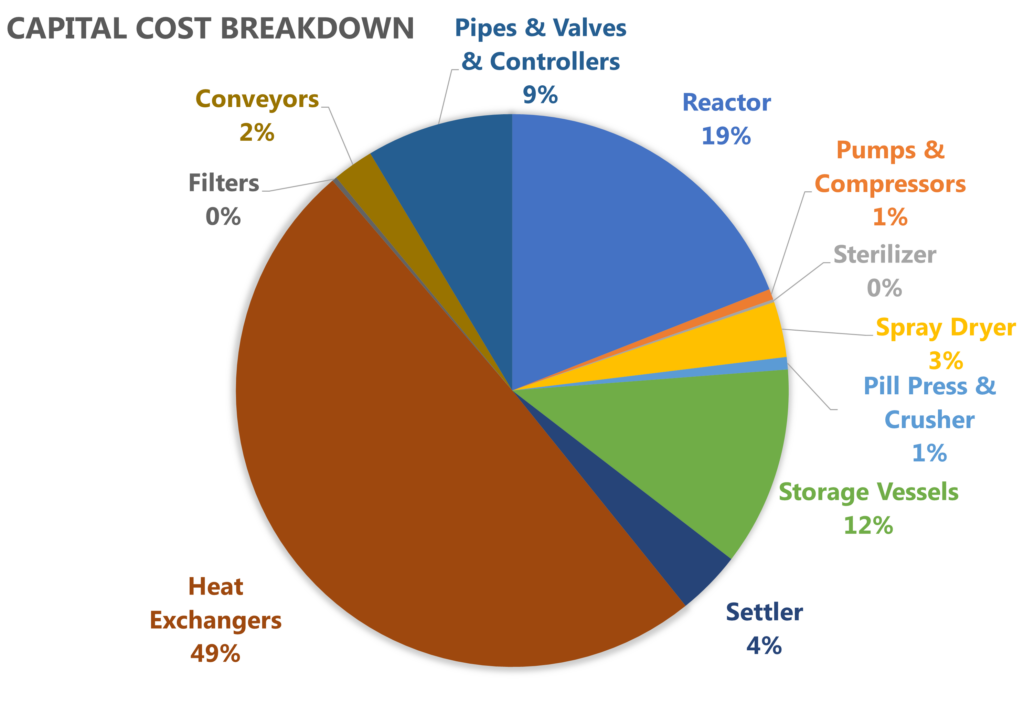
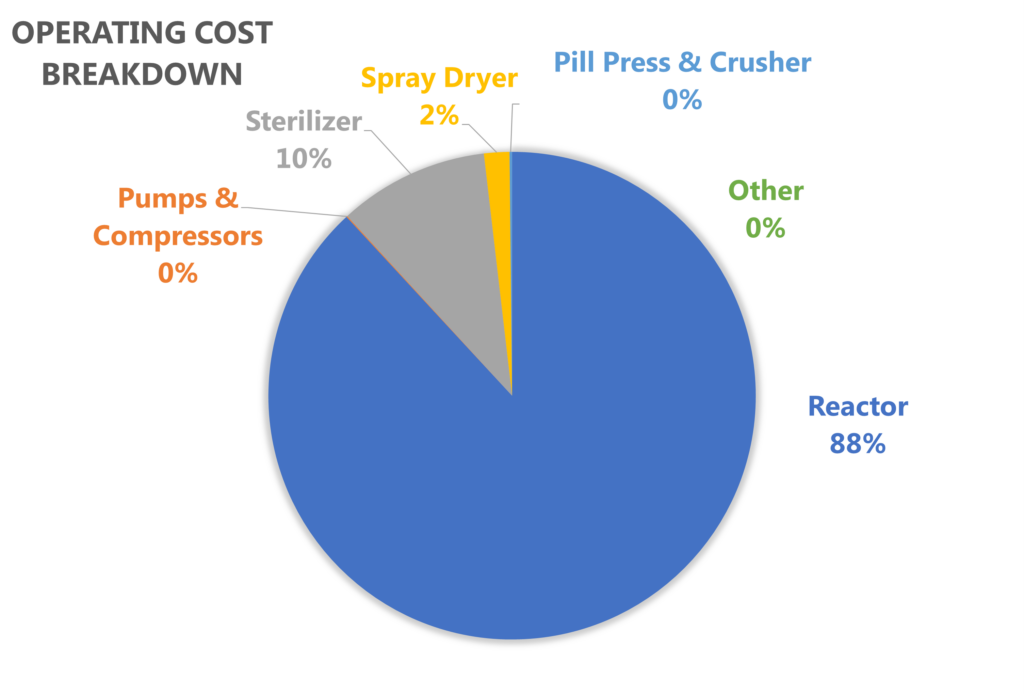
Profitability Analysis
The profitability analysis includes calculating the gross profit margin and the net profit margin. The gross profit margin shows the percentage of sales revenue a company keeps after covering all operation costs and the net profit margin shows the profit generated for every $1 in sales.
The gross profit margin was calculated to be 73.2%. If the gross profit margin is higher than 65%, this is considered to be healthy, meaning that this company has more money to pay for indirect and other costs. The net profit margin was calculated to be 54.2%. If the net profit margin is over 10%, this is considered to be a high value, meaning that this company will be able to effectively control its costs and provide goods or services at a price higher than its costs. Since both the gross profit margin and the net profit margin are above the recommended values, this design is considered to be economically feasible.
Additionally, the cash flow shows that this company will start making a profit by 2025 and by the end of the predicted lifespan of the equipment, will have a net positive cash flow of over $7.8 million dollars, with an annual income of $580 thousand per year.
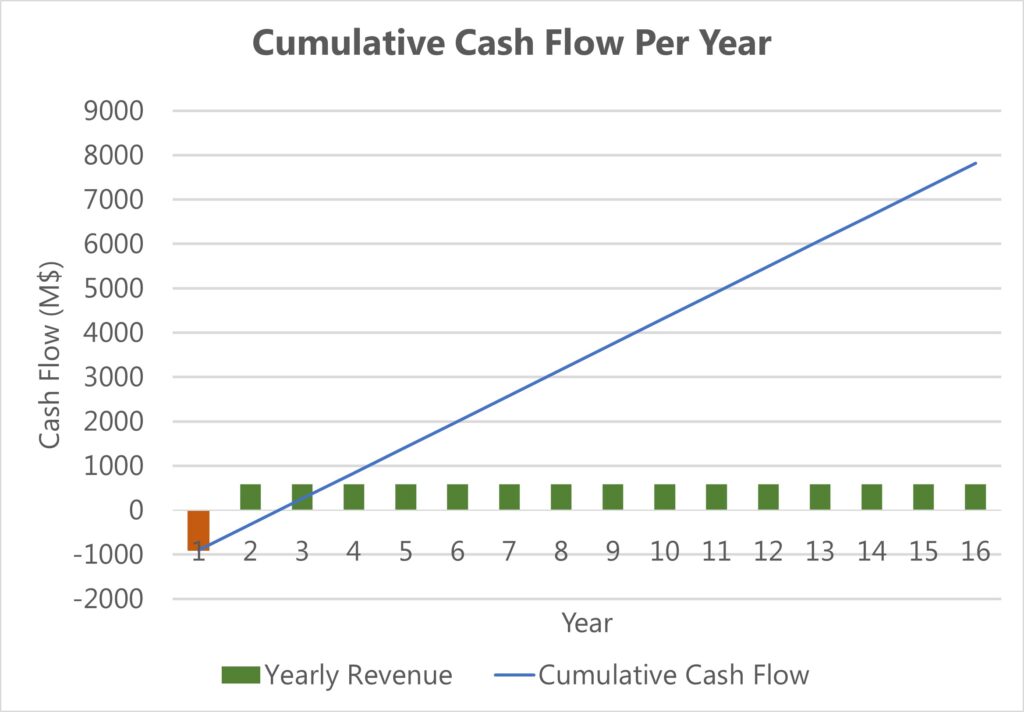
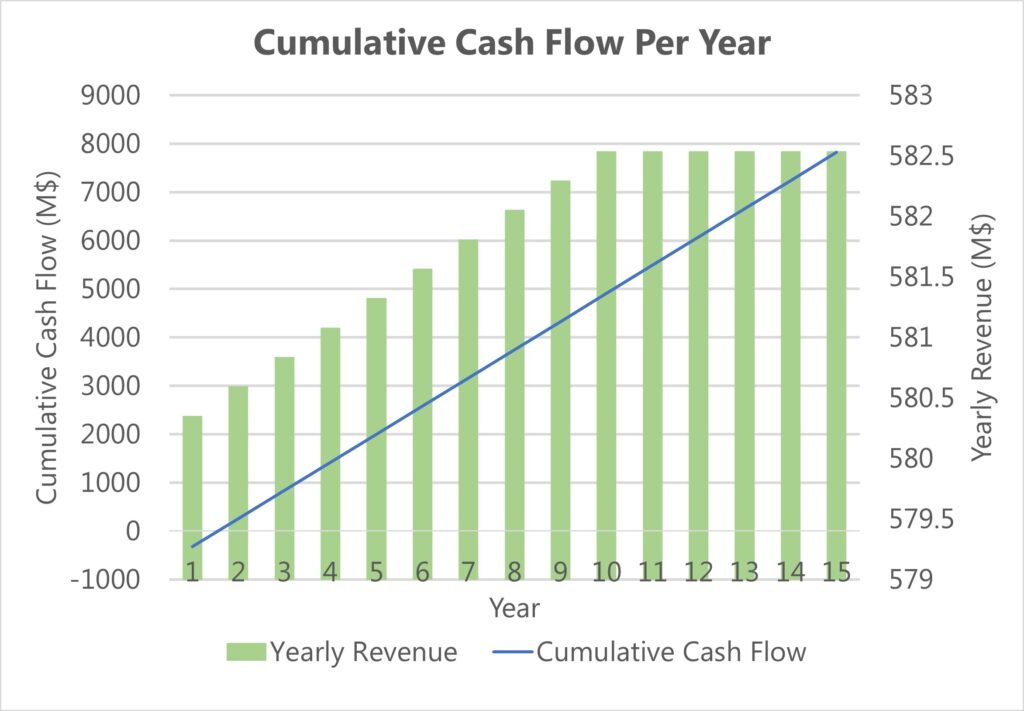
Sensitivity Analysis
As shown by the “Optimizing Reactor Conversion” plot in the previous section, a 90% reactor conversion was selected because the growth rate of Chlorella vulgaris increases significantly at this point, which would require far too many reactors in our process. A sensitivity economic analysis of the process is shown below to compare the nominal 90% conversion (mid-point on the plot) to conversions of 50% (much lower than the optimal conversion) and 95% (slightly higher than the optimal conversion). It can be seen that at 50% conversion, while costs are lower, so are the revenues and at 95% conversion, the increased costs outweigh the increased revenues.
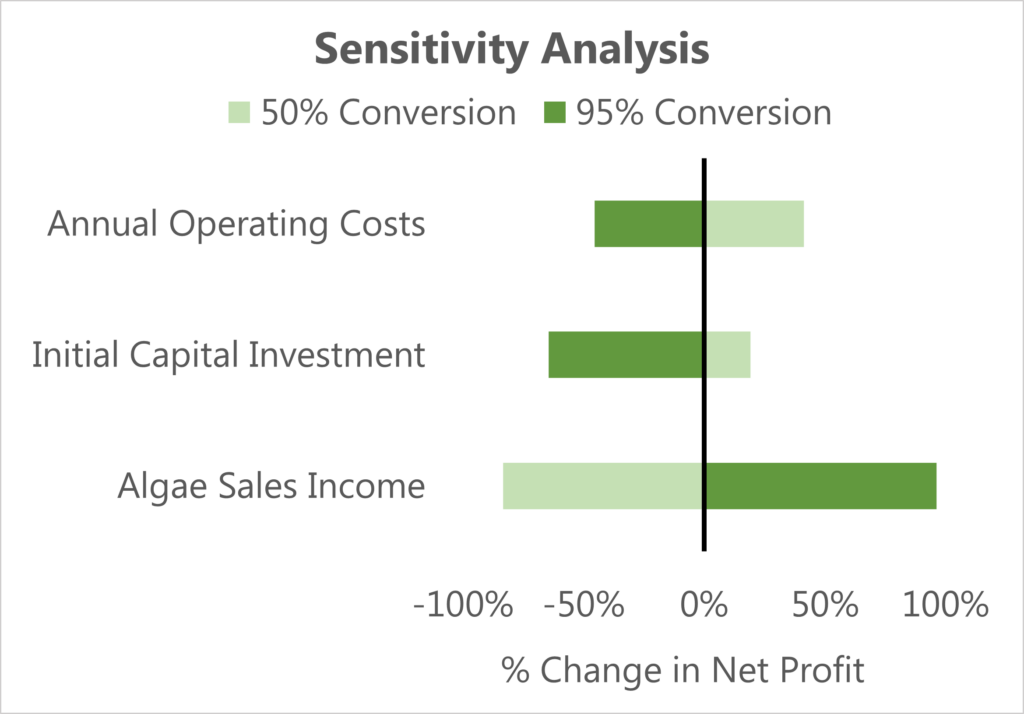
SAFETY CONSIDERATIONS OF OUR DESIGN
Consumer Product Considerations
The Chlorella vulgaris tablets produced in this process are natural health products, therefore the production of these tablets must abide by the Natural Health Products Regulations which are a series of regulations under the Food and Drugs Act of Canada (Health Canada, n.d.). Fahr Beer will be required to apply and be granted a product and site license, before beginning the production process. The product produced in this process will contain only Chlorella vulgaris without any additives, or fillers. The tablet products will contain 500mg of Chlorella vulgaris and the recommended use will be to consume four tablets a day with a meal. Once Fahr Beer receives their license, they will be issued an eight-digit Natural Product Number (NPN), that needs to be displayed on the label of the superfood tablets. Apart from the NPN, the label will include the product’s name, ingredients, weight, recommended use, and instructions to store the container in a cool, dry place, away from heat or light sources. A warning on the label will indicate that the product is not intended for children under the age of 18 and that women who are pregnant or people with any known medical conditions should consult a licensed physician before consuming this product.
Process Design for Food Safety Regulations
In order to prevent any contamination in this process the heat exchangers, storage tanks, pumps, and settler units will be constructed using stainless steel and carbon steel, ensuring that surfaces are smooth, non-corrosive, non-absorbent, and non-toxic, to prevent pitting, cracks or crevices and the surfaces will be able to withstand repeated cleaning and sanitation (Canadian Food Inspection Agency, 2014). In the current process design, there are no cross-connections between potable and non-potable water supplies. All hoses, taps, and valves are designed to prevent back-flow or back siphonage. Recirculated water from condensate or for re-cooling will be monitored and maintained to prevent equipment corrosion. For any heating of equipment or heat exchanger units, the potability of steam that is in direct contact with food or food contact surfaces is controlled to prevent product contamination. The steam supply is sourced from potable water and meets operational requirements, furthermore, traps will be installed as necessary to ensure sufficient removal of condensate and other foreign contaminants.
ACKNOWLEDGEMENTS
The Algaeneering team wants to thank the many people who helped us with our project throughout the Fall 2021 and Winter 2022 semesters:
Firstly, our chemical engineering process design course professor and team supervisor, Dr. De la Hoz Siegler who met with us weekly and guided us through the design process with patience and great expertise.
Next, our client and industry mentor, Jochen Fahr who provided specialized advising on the Fahr brewery process at every step of the way.
As well, Joenel Alacantra and Erin Brown for lending their biological knowledge on algae cultivation and growth, and supplying our starting Chlorella vulgaris sample. Finally, we would like to thank Cidgem Demirkaya for mentoring the team in the laboratory as we built our prototype reactor and learned how to inoculate our Chlorella vulgaris medium.
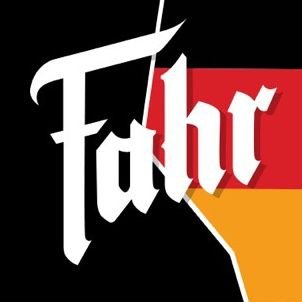
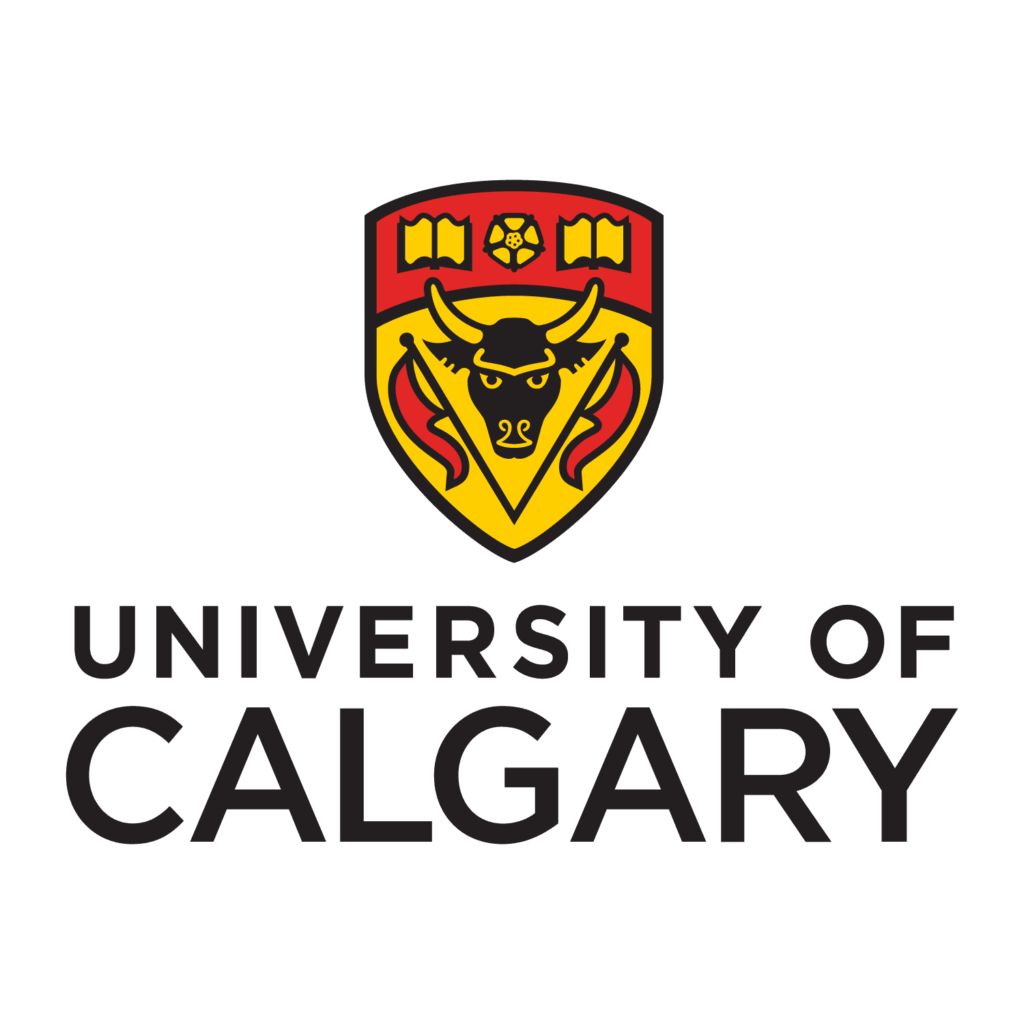
Our photo gallery
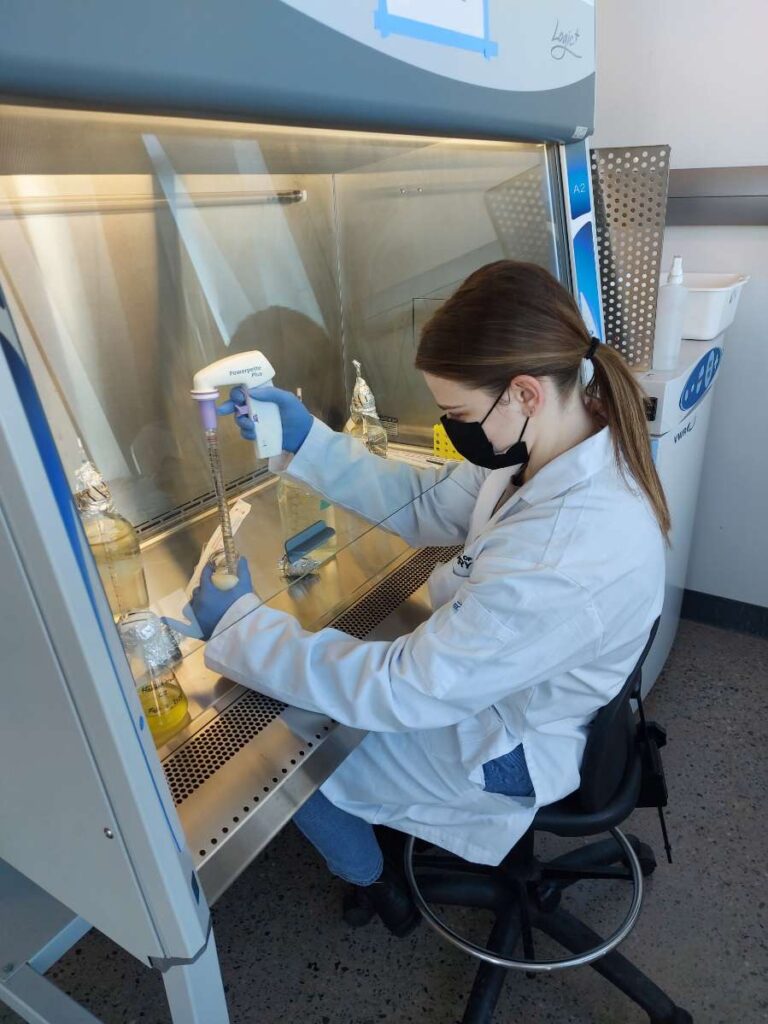
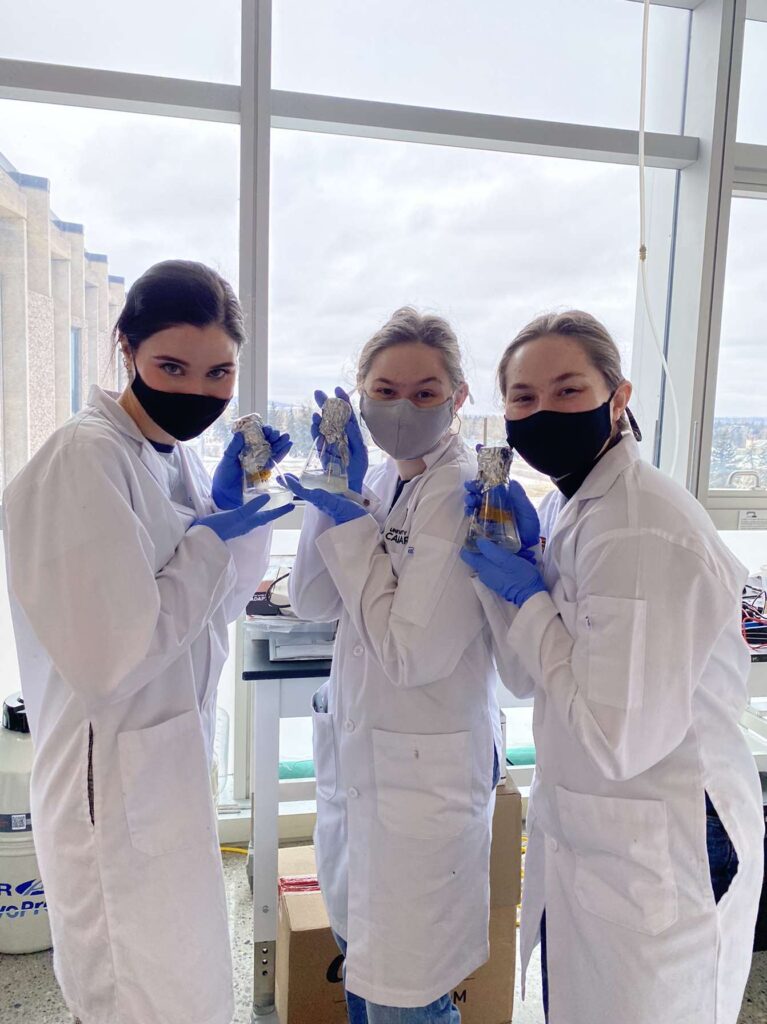
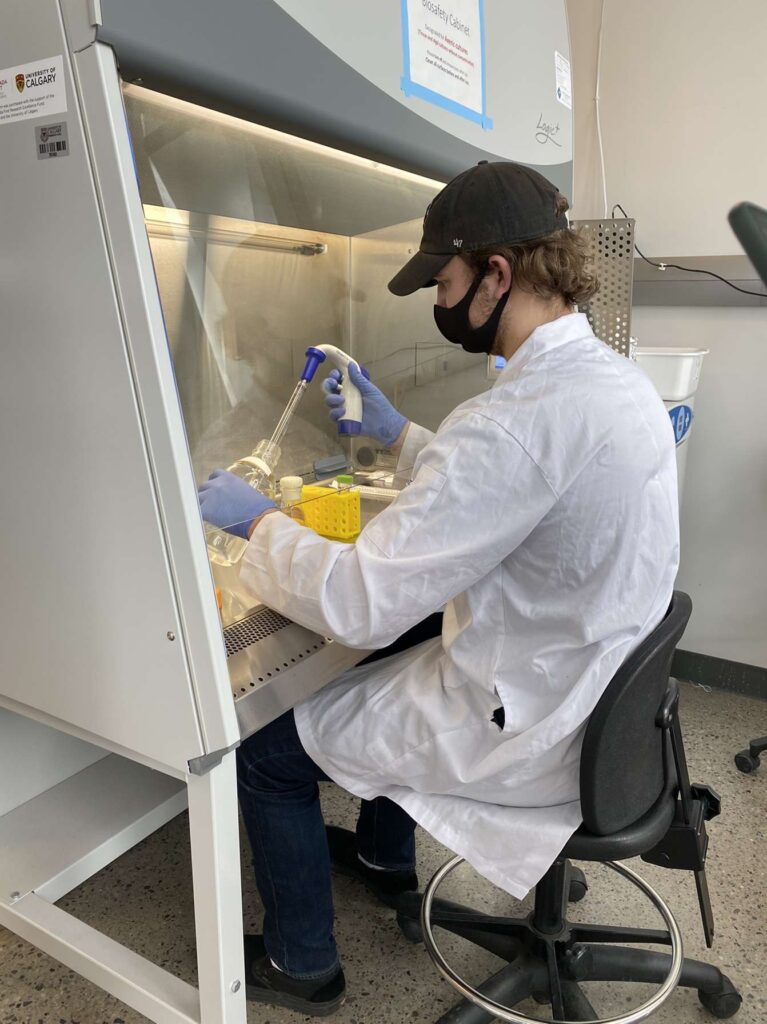
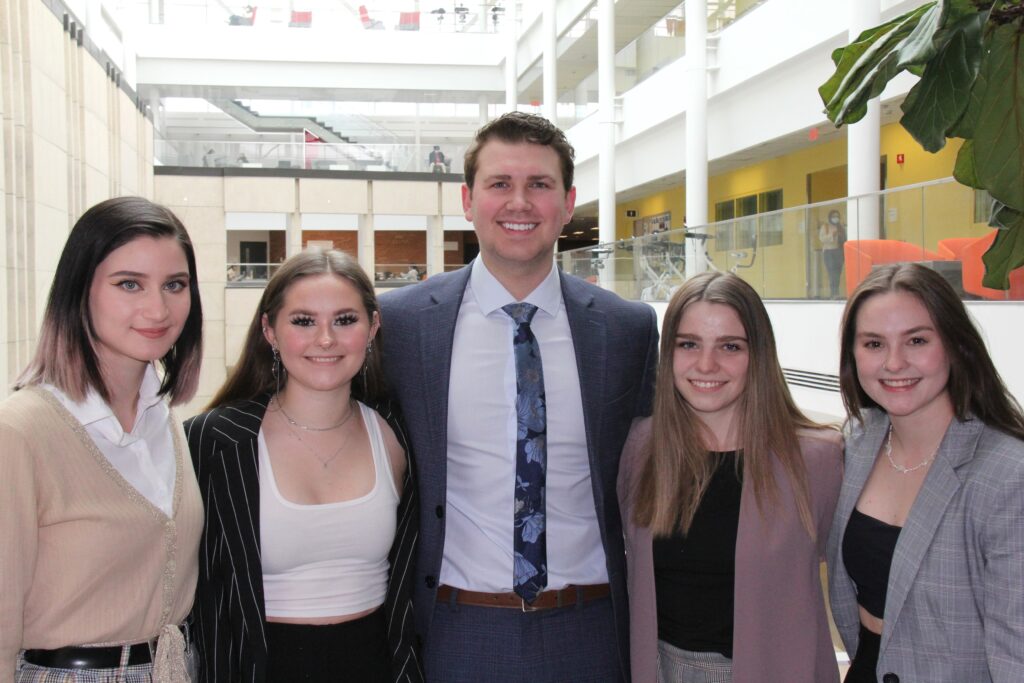
References
Works Cited
[1] Bennett, C.; Kistler, R.; Lestina, T.; King, D. Improving Heat Exchanger Designs. Chemical Engineering Progress 2007, 103, 40-45.
[2] Benz, G. T. Bioreactor Design for Chemical Engineers. American Institute of Chemical Engineers (AIChE) 2011, 21–26.
[3] Doran, P. M. In Bioprocess Engineering Principles; Academic Press: London, 2003; pp 377–386.
[4] Carbon Taxes & Rebates Explained (Province by Province), 2021. Canada Drives. https://www.canadadrives.ca/ (accessed Oct 8, 2021).
[5] Fact.Mr. Algae Supplements Market Forecast, Trend Analysis & Competition Tracking – Global Market Insights 2019 to 2027. Algae Supplements Market 2019. https://www.factmr.com/report/2546/algae-supplements-market (accessed Dec 1, 2021).
[6] Fogler, S. H. Elements of Chemical Reaction Engineering, 5th ed.; Pearson Education, Inc.: Kendallville, Indiana, 2016.
[7] Government of Canada. The Low Carbon Economy Leadership Fund, 2018 https://www.canada.ca/en/environment-climate-change/news/2018/04/low-carbon-economy-leadership-fund.html (accessed Dec 10, 2021).
[8] IndustryARC. North American Algae Protein Market – Forecast(2021 – 2026) 2020. https://www.industryarc.com/Research/North-American-Algae-Protein-Market-Research509543 (accessed Dec 1, 2021)
[9] Lin, J. 8 steps to successful profitability analysis https://www.centage.com/8-steps-for-successful-profitability-analysis/ (accessed Dec 2, 2021).
[10] McCabe, W. L., Smith, J. C., & Harriott, P. (1993). Chapter 30 – Mechanical Separations. In Unit Operations of Chemical Engineering (5th ed., pp. 1003–1020). essay, McGraw-Hill Education.
[11] Pegallapati, A. K.; Nirmalakhandan, N. Modeling algal growth in bubble columns under sparging with CO2-Enriched Air. Bioresource Technology 2012, 124, 137–145.
[12] Perry, R. H., & Green, D. W. (2019). Section 18.7 – Filtration. In Perry’s Chemical Engineers’ Handbook (9th ed.). essay, McGraw-Hill Education.
[13] Ranade, V., Kulkarni, A., & Rampure, M. Hydrodynamics of Bubble Column Reactors at High Gas Velocity: Experiments and Computational Fluid Dynamics (CFD) Simulations https://pubs.acs.org/doi/pdf/10.1021/ie070079h (accessed Mar 5, 2022).
[14] Seider, W. D.; Seader, J. D.; Lewin, D. R.; Seider, W. D. Product and process design principles: Synthesis, analysis, and evaluation; John Wiley & Sons: New York, 2004.
[15] Walas, S. M. Chemical Process Equipment: Selection and Design; BH, Butterworth-Heinemann, an imprint of Elsevier: Amsterdam, 2012.
[16] Yun, Y.-S.; Park, J. Attenuation of monochromatic and polychromatic lights in Chlorella vulgaris suspensions – applied microbiology and biotechnology https://link.springer.com/article/10.1007/s002530100639 (accessed Mar 5, 2022).
Design Specifications

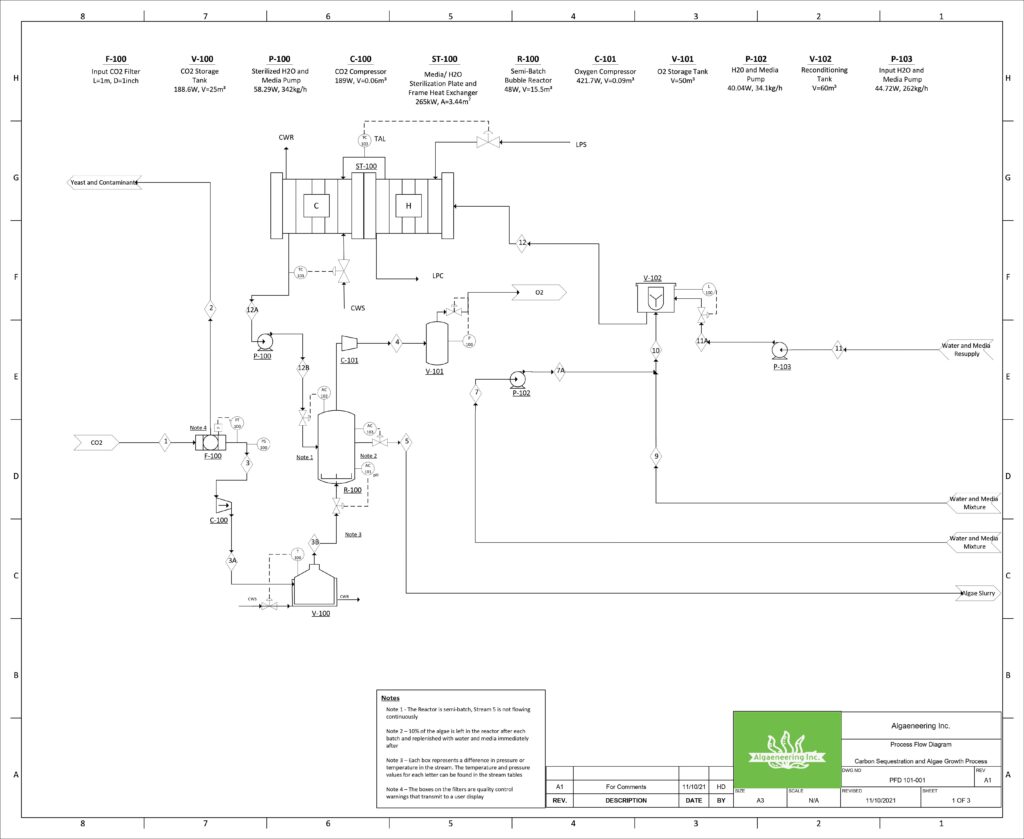

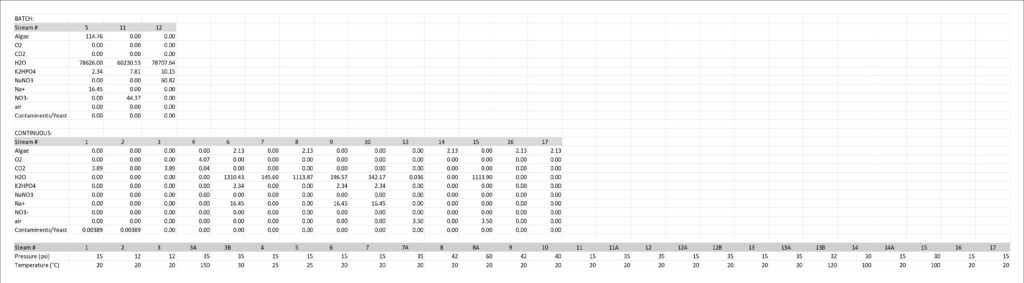
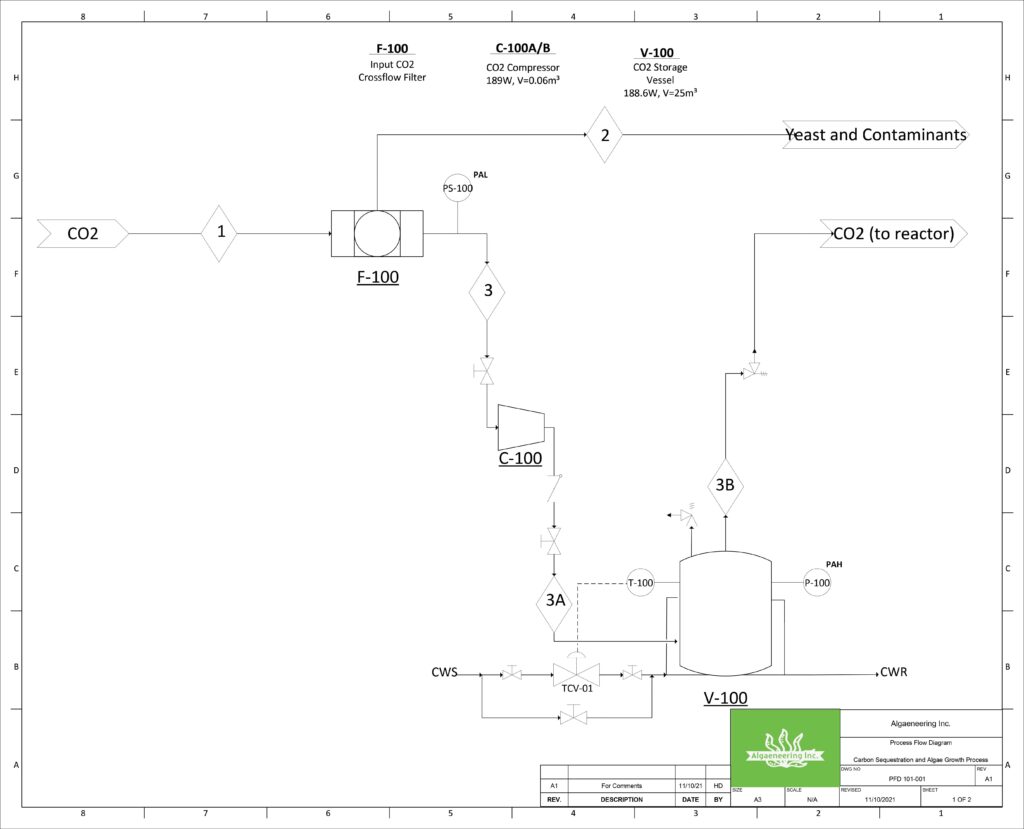

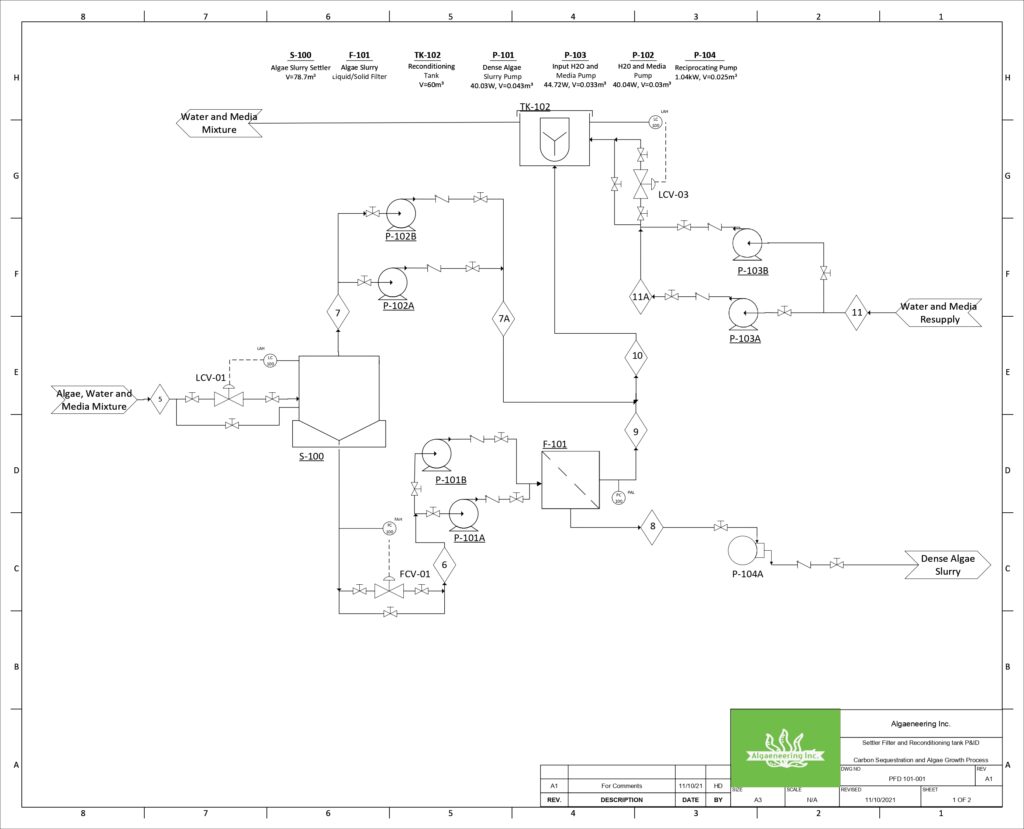

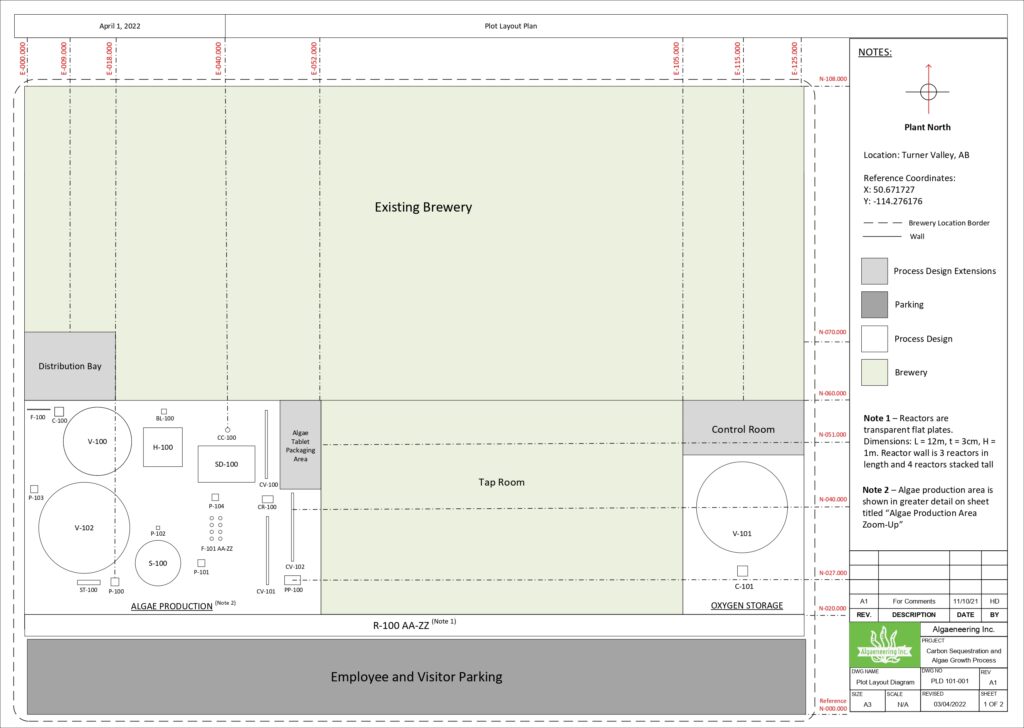

