Project Category: Chemical
Join our presentation
About our project

Blue energy is a renewable and sustainable source of energy, which has the potential to replace fossil fuels in the near future. Progressive engineering introduces the energy extraction process by using hydrogel technology.
Meet our team members

Details about our design
HOW OUR DESIGN ADDRESSES PRACTICAL ISSUES
Fossil fuels are used globally to provide energy. They have several disadvantages: emitting greenhouse gases, carbon emissions, depleting a finite resource, and relying on the exports of specific regions.
One of the main benefits of blue energy is that the only residual element generated from its use is salt water. No emissions of any sort are released into the atmosphere. Its use could mark an unbeatable milestone in sustainability. Blue energy utilizes the salinity gradient between fresh and salty water, to harness the energy produced when they mix. It is estimated that 1.4-2.6 TW of energy can be recovered from salinity gradient power to fulfill the global energy demand of 2 TW.
WHAT MAKES OUR DESIGN INNOVATIVE
Hydrogels have never been utilized at an industrial scale to produce power, this plant design would be the first of its kind anywhere in the world.
WHAT MAKES OUR DESIGN SOLUTION EFFECTIVE
Progressive Engineering is designing a hydrogel plant in Mackenzie County. This technology utilizes the swelling of polyacrylic acid hydrogels in the presence of freshwater and shrinking from exposure to high salinity water. As freshwater flows through hydrogels, electrostatic repulsion causes the hydrogel to expand, allowing it to take in large quantities of water. When high salinity water flows through the hydrogel, the electrostatic repulsion is reduced shrinking the size of the hydrogel, with an additional salting out effect on the polymer network making the polymer shrink to its original size. The swelling of the hydrogels is used to lift a weight or compress a piston to perform mechanical work. This is one of the only solutions to produce mechanical work from hydrogel expansion/contraction.
DETAILED DESIGN
The proposed design requires several specialized units. The detailed design of the main process units can be seen below.
Fresh and Saltwater Pumps
Two centrifugal pumps carrying fresh and saltwater streams with flow rates of 22.5 L/s and 13.51 L/s, respectively, are each connected to a 10-meter pipe attached to a valve that regulates the flow. The velocities were calculated for both streams (2.76 m/s for salt water, 2.74 m/s for fresh water). Pump sizing involved matching the flow and pressure rating of the pump with the flowrate and pressure required for the process. Achieving this flowrate requires a pump that can generate a pressure high enough to overcome the hydraulic resistance. To estimate the pump sizing, the system head, as well as the power required to operate both pumps were calculated, resulting in pump head of 1.5 m for fresh and 1.7 m for salt water with power consumption of 0.49 kW for fresh water and 0.32 kW for saltwater pumps.
Hydrogel Piston
The hydrogel piston will be built out of glass fiber reinforced epoxy, with a bore size of 0.69 m and a height of 15 m. Ten pistons will be utilized, with a stroke length of 14.7 m. Each piston will be filled with 27 kg of poly4-styrene sulfonic acid co-maleic acid interpenetrated in a polyacrylic acid network (PAA/PSSA-MA1.5) and will be used to produce ~1 kW of energy. The piston arm will be 14.7 m long with a radius of 0.10 m, and base of 0.69 m, thickness of 0.1 m, with a weight of 908 kg. The hydrogel piston will accumulate freshwater, and as saltwater is pumped through, the fresh water will be released and mixed with saltwater.
Hydraulic Piston Cylinder
Single-acting steel cylinder was chosen for the process. This design was based on the swelling basis of hydrogels. The cycle time was taken to be 15 minutes. 250 kg of silicone oil will be used to circulate in the hydraulic system that will increase the pressure in the compressed air tank. The temperature of silicone oil was assumed to be the ambient temperature of 25 oC. Efficiency of the hydraulic piston cylinder was found to be 95%. Using moment balance over hydrogel and hydraulic pistons, the force on the hydraulic piston was found to be 2260.6 N. The volume of silicone oil was calculated to be 0.24 m3 followed by calculation of the mass of silicone oil of 231.12 kg. Bore radius was estimated to be 0.8 m with piston radius of 0.4 m and stroke length of 1 m.
Hydraulic Pump
A rotary positive displacement pump was chosen because of its ability to pump high viscosity fluid. To find the power required to operate the pump, volumetric flow rate was first calculated. By using a cycle time of 900 seconds and previously calculated volume of silicone oil (0.24 m3), the volumetric flowrate was calculated to be 0.000267 m3/s. With a change of pressure of 3.94 MPa, pump power was found to be 1.503 kW.
Compressed Air Tank
Air is assumed to have ideal gas behaviour under the operating conditions. Using energy efficiency, energy transferred to hydraulic piston, we calculated the energy transferred to the hydraulic piston is equal to 3.42 MJ per cycle. Calculated pressures at the start and end of the cycle is equal to 12.46 MPa and 16.4 MPa, respectively. The volume of the tank was found to be 1 m3.
Turbine-Generator
The turbine will use propellers made of fiberglass that are 0.40 meters in diameter. The height of each blade is 0.04 meters and will rotate at 1500 Revolutions Per Minute (RPM). The inlet pressure of the turbine is 108.325 kPa through a nozzle with a width of 0.118 meters. The turbine will discharge to atmospheric pressure, which is calculated to be 95.4127 kPa at the average elevation of the proposed plant location. The rotating shaft of the turbine will be connected to a generator producing 1333.3 Watts of electricity from a dry air mass flow rate of 0.843 kilograms per second.
ENVIRONMENTAL CONSIDERATION
This hydrogel plant does not produce any carbon emissions. The plant uses wastewater to reduce the environmental impact of the mining and other fossil fuel industries. The plant will be utilizing high salinity wastewater from a reservoir sourced from the industrial mining industry. The water contains various saline concentrations. This plant will produce 1590m3/day of wastewater that needs to be treated before it can be returned to the environment. Progressive Engineering has evaluated that electrodialysis is the ideal wastewater treatment to remove the various metal ions, present in the water. It can be run at any time of the day and removes salt ions from the solution. An external plant will be contracted to treat the wastewater.
FEASIBILITY OF OUR DESIGN SOLUTION
Total operating expenses in the first full year of plant operation is found to be $11.6 million in 2024 while Total operating expenses in the first full year of plant operation is found to be $11.6 million in 2024 while producing 16574.32 kWh of electricity per year. The main source of revenue for this project is the sale of electricity generated by the plant. The energy being generated is consumed by the plant itself. The current challenges facing hydrogels are related to the amount of energy that can be captured, and low efficiency of the hydrogels. We recommend removing the hydraulic pump and piston to reduce the energy consumption of the plant. In its current state, the plant is consuming more energy than it is producing, leading to a design that is not feasible.
Partners and mentors
We want to thank the many people who helped us with this project. Our chemical engineering project supervisor, Dr. Qingye Lu guided us through the process with infinite patience and great advice. Additionally, our consultation with chemical engineering professor Dr. Michael Foley, was invaluable.
Our photo gallery
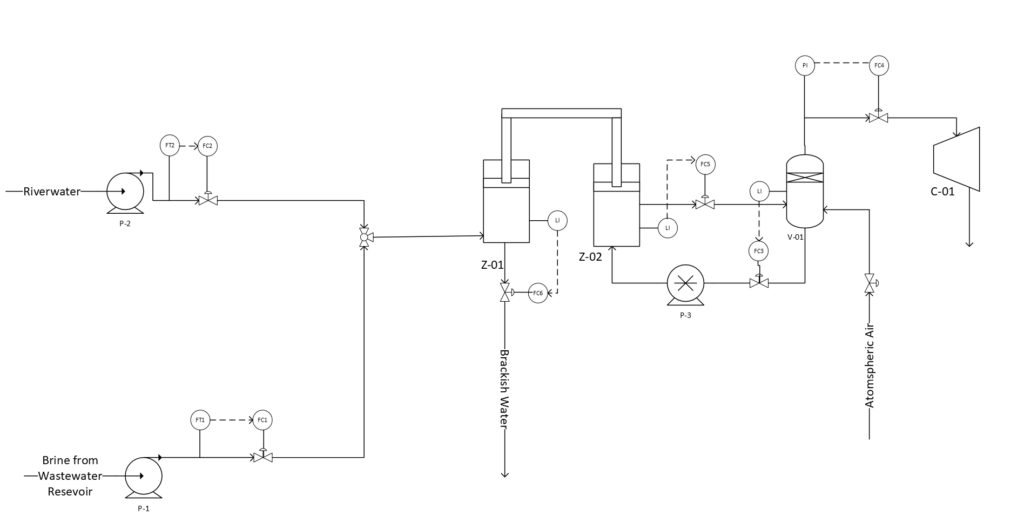
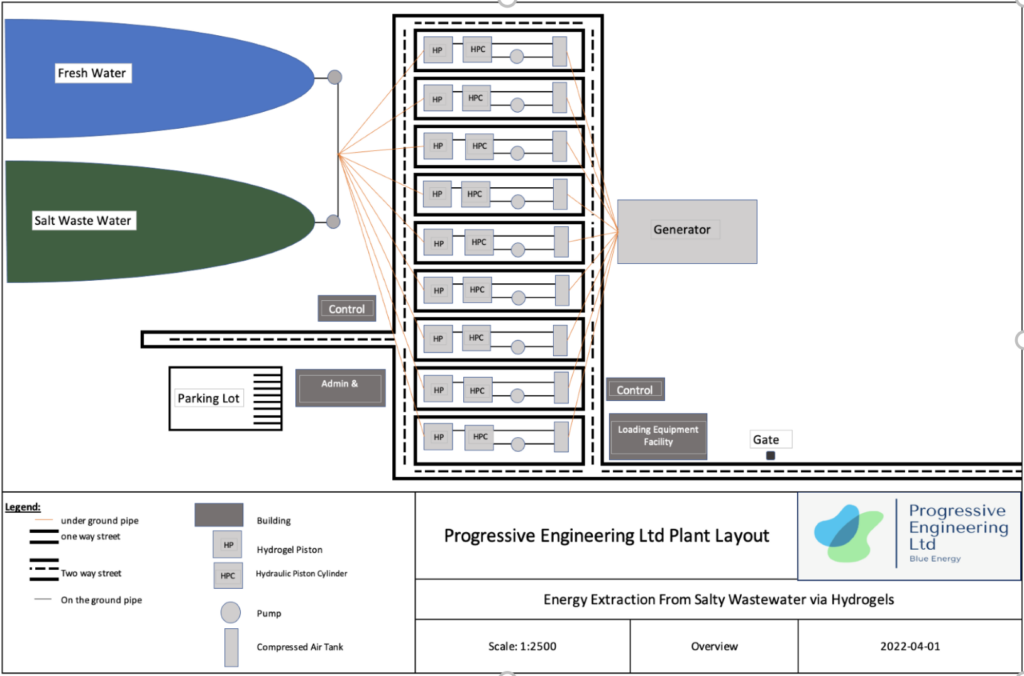
References
- David, O. C., Gorri, D., Nijmeijer, K., Ortiz, I. & Urtiaga, A. Hydrogen separation from multicomponent gas mixtures containing CO, N 2 and CO 2 using Matrimid ® asymmetric hollow fiber membranes. J. Memb. Sci. 419–420, 49–56 (2012).
- Bui, T. Q. et al. Salinity Gradient Energy from Expansion and Contraction of Poly(allylamine hydrochloride) Hydrogels. ACS Appl. Mater. Interfaces 10, 22218–22225 (2018).
- Zhu, X., Yang, W., Hatzell, M. C. & Logan, B. E. Energy recovery from solutions with different salinities based on swelling and shrinking of hydrogels. Environ. Sci. Technol. 48, 7157–7163 (2014).
- Bui, T. Q., Cao, V. D., Wang, W. & Kjøniksen, A. L. Recovered Energy from Salinity Gradients Utilizing Various Poly(Acrylic Acid)-Based Hydrogels. (2021).