Project Category: Chemical

Lithium Extraction from Oil and Gas Brine
Join us on Zoom! April.5th 9:30am – 1:30pm
About Our Project
With global demand for lithium resources rapidly outpacing supply due to the needs of the growing electrification of the transportation industry, sustainable and feasible alternative lithium extraction and processing must be developed.
Our process aims to extract lithium resources from Devonian-aged formation brines in Alberta, where presently no active commercial-scale production exists due to the challenging nature of lithium ion concentrations. According to the Alberta Energy Regulator, Alberta possesses an inferred 746 thousand tonnes of lithium carbonate equivalent throughout just two of the province’s vast geological formations, typically exploited for oil and gas production. With global demand expected to reach 1.597 million tonnes per year by 2030 for lithium ion battery demand alone, Alberta is poised to become a leading producer of lithium in the near future.
Our design solution, utilizing direct lithium extraction technology, aims to address the existing challenges of not only Alberta’s lithium resources, but also present-day commercialized lithium production methods to directly target the lithium ion battery market, and further innovate the industry as a whole.
Forecasted LCE Demand for Li-ion Batteries by Application
Meet Our Team Members
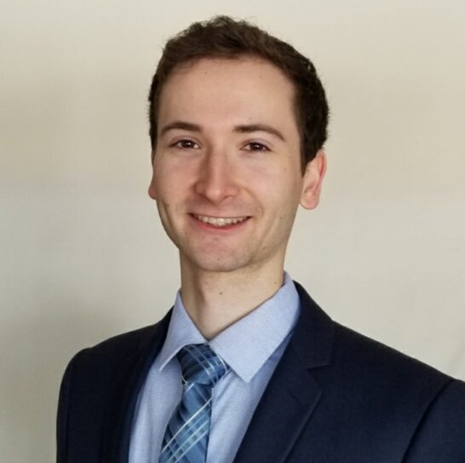

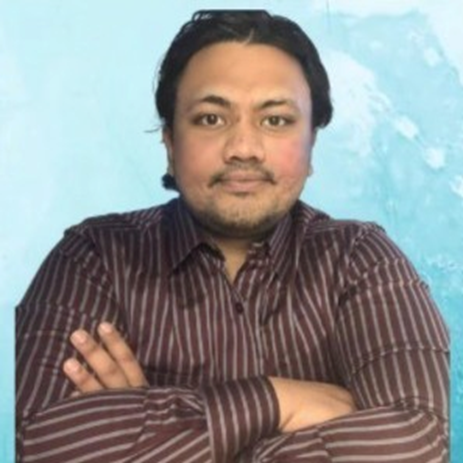

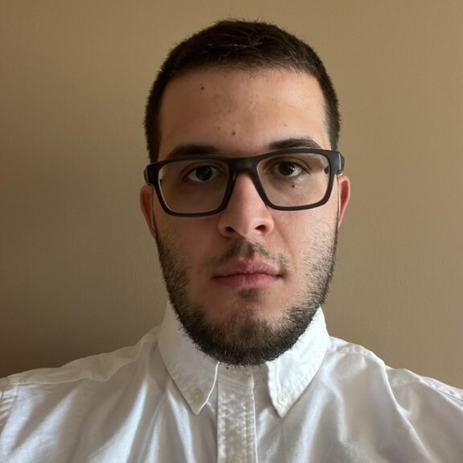
Details About Our Design
HOW OUR DESIGN ADDRESSES PRACTICAL ISSUES
Presently, the vast majority of lithium is produced by hard-rock mining operations predominantly in Australia, accounting for approximately 46% of global lithium supply, and 31% from brine in South America concentrated through the use of large-scale evaporation ponds, as of 2020.
These existing industry practices carry significant challenges, particularly with the open-air evaporation ponds in South America, such as geographic constraints impacting the feasibility of using ponds, large land usage requirements, high rates of underground water loss in regions with limited water resources, as well as long production lead times – typically 12 to 18 months from start-up – making it difficult to rapidly respond to market pressures.
Likewise, the evaporation ponds are not viable to implement for Alberta’s brines due to the province’s cooler and wetter climate, and relatively low lithium ion concentrations of approximately 75 parts per million (ppm) compared with up to 1000 ppm in South America, thereby requiring significantly more volumes of water to achieve comparable lithium production rates.
Brine evaporation pools of Atacama salt flats, Chile.
As such, our design includes a large-scale centralized processing facility targeting Alberta’s brine formations, capable of processing 105000 m3/day of raw brine feed and producing 6700 tonnes per year of lithium hydroxide monohydrate, a battery-grade lithium compound, while utilizing ion-exchange technology to selectively extract the lithium ions, further allowing us to return a significant portion of the lithium depleted waste water back to Alberta’s confined aquifers.
WHAT MAKES OUR DESIGN INNOVATIVE
In addition to directly extracting lithium-bearing brine and subsequent ability to dispose of waste materials through the use of producing and injection wells, our design solution has several key innovations that further address the present industry challenges and improve upon the feasibility of our process.
Recovery of Valuable Co-products
Through the use of our divalent ion processing units, our design includes the separation and recovery of valuable strontium metal also found within the raw brine stream, further allowing us to add additional value creation within our processing facility.
Ion Exchange for the Direct extraction of Lithium Ions
To address the challenging lithium concentration found in Alberta’s brine resources, our ion exchange unit utilizes the titanic acid resin (H2TiO3) which has multiple innovative features proven with laboratory experiment validations:
- Titanic acid was proven to be capable of efficiently adsorbing lithium ions from the brine containing competitive monovalent cations such as sodium and potassium in extremely large excess.
- Resin takes advantage of size effect (variation in ionic radius) that result in preference for Li+ : Li+ ≫ Na+, K+, Mg2+, Ca2+ (originates from size effect)
- H2TiO3 have over 98% regenerative capacity, reducing resin replacement frequency and costs
Reverse Osmosis for Solution Concentrating
Following the ion exchange unit, the lithium-rich solution must be further concentrated before additional processing. By utilizing reverse osmosis membrane technology, our process generates valuable clean water that can be sold and used for agriculture purposes from the brine that would typically be unusable for direct commercial applications due to the high salt contents. This design feature is in contrast to the typical ponds used in South America which waste up to 500,000 gallons of valuable water resources per one tonne of lithium produced due to the open-air evaporation. Likewise, by using reverse osmosis with electric driven pumps in large scale, compared to typical industrial alternatives such as evaporation using steam generated via natural gas, our design solution can take advantage of Alberta’s decreasing carbon intensity of electricity thereby minimizing future carbon emissions.
WHAT MAKES OUR DESIGN SOLUTION EFFECTIVE
In order to support the effectiveness of our design at recovering lithium, while both minimizing environmental impacts and remaining economically feasible, our team implemented a variety of design improvements and solutions.
Typical industry practices of open-air evaporation for lithium salt recovery are time consuming, damaging to local ecology, and very much weather dependent. Our centralized processing facility utilizing direct lithium extraction with highly selective resins enables continuous operations independent of weather and dramatically reduces risk of contamination to surrounding areas, while also producing viable quantities of usable water resources. Moreover, recovery of valuable strontium from what would otherwise be waste supports the value creation of our process. Likewise, selective ion exchange allows for further processes towards battery-grade lithium hydroxide monohydrate which sells at a premium market price compared to lower grade lithium salts typically produced from evaporation ponds.
Hydrogen Sulfide Removal
When extracting brines from formations typically used for hydrocarbon production, approximately 300 ppm of H2S is contained with the brine. H2S is removed through the use of a stripping column with an diethanolamine (DEA) treating process, with further brine stream polishing through oxidation to ensure safe facility operations and meet regulatory requirements. H2S can then be injected with wastewater streams back to the geological formation.
Premium Battery-grade Lithium Production
With the use of selective ion exchange resins, lithium-rich stream purities enable further processing to create lithium hydroxide monohydrate, maximizing the value of Alberta’s lithium resources. Through a combination of reactors and washing treatments designed to first precipitate a raw lithium carbonate using Na2CO3 reagent, and then further processing with Ca(OH)2 to a highly pure LiOH solution. Final evaporative crystallization volume requirements are significantly reduced, along with subsequent energy demands, all the while maintaining greater than 99.3% final product purity and a relatively uniform crystal size distribution needed for the lithium-ion battery market.
Heat Recovery
The implementation of waste heat recovery reduces overall process requirements, improving operating costs while also decreasing utility demand, supporting process sustainability. For example, the DLE Ion exchanger used a closed loop system, reducing operations cost by $8,237.5/regeneration cycle and Natural Gas usage by 516.4 MCF/regeneration cycle. Similarly, brine needs to be cooled in order to precipitate the strontium hydroxide. Immediately after this the brine is reheated for the second reaction, the cold brine is run through a heat exchanger with the previous hot brine that needs to be cooled. This allows for the recovery of a large portion of the energy and in turn a large reduction in the utilities required.
HOW WE VALIDATED OUR DESIGN SOLUTION
There are several challenges associated with simulating and validating design results for a DLE Ion Exchange plant. Given that direct lithium extraction technology is yet to be implemented on an industrial large scale, it is no surprise that there are few simulating softwares to model the process as a whole. Our team identified the need to model using a combination of literature research, company patents, MATLAB, and hand calculations for design and validation. When it came to choosing DLE technology over alternative evaporation and membrane methods for lithium extraction, we completed a Kepner-Tregoe method analysis comparing each of the three methods to quantify the desired approach.
The fixed input variable was 105,000 m3/day of raw brine feed, which was used to design operating parameters and output (production) rate. The brine stream has 20+ different chemical components, which contain trace concentrations of various metals that are difficult to account for with adequate degree of accuracy. Another challenge was the dynamic nature of brine feed composition that will not remain constant as fluid is being pumped from varying geological formations. As such, average water sampling data completed between 2017-2020 by E3 Metals Corp. was utilized and assumed to be consistent over the course of plant operation.
Literature review included latest technology and resin findings to identify a suitable adsorptive resin that will work on low Li+ concentration brine solutions. H2TiO3 was especially selective on lithium in brine solutions with high calcium and magnesium but low lithium concentrations, comparable to our feed. The figure to the right shows variation of Li+ concentration with time, inside the ion exchanger, created with MS Excel.
To the left is an example of a reactor modeled as a CSTR with Jacket dynamics to maintain the temperature while the reaction is taking place completed with MATLAB. The assumption for this reaction to be able to model it as a CSTR is good mixing to have uniform composition throughout the reactor, as well as the exit stream having the same composition as in the tank. The brine is contacted with calcium hydroxide to produce strontium hydroxide which can be precipitated out of the stream by dropping the temperature. Using plots of this reaction at different temperatures over time, the reaction kinetics were able to be determined. After the precipitation of strontium the brine is contacted with sodium carbonate in order to facilitate the precipitation of the rest of the strontium as well as the existing and added calcium. This effectively removes 90% of the divalent ions from the system and greatly improves the lithium adsorption prior to the ion exchanger.
FEASIBILITY OF OUR DESIGN SOLUTION
The selling price per metric tonne of lithium was evaluated at $19,000 was considered the baseline for this project. This value was used to determine the economic feasibility of the project, however the price of lithium has since increased significantly to approximately $65,000 per metric tonne as a result of post-pandemic supply and demand factors, further validating the need for diverse stable supplies of lithium resources globally. The figure to the right shows the selling price of lithium over the past 20 years, including the next 10 years of what the selling price is projected to be.
The economics of this project have been calculated at an initial capital cost of approximately $143 million. The operating costs of this project are calculated at approximately $132 million, and the total revenue before tax is calculated at approximately $170 million. With all of this information, the post tax revenue of this project stands at $20 million annually with a payback period of 8 years assuming 2 years initially to build the plant.
To the left is a Strauss Plot for a sensitivity analysis. The plot considered a 20% change positive and negative for variables that affect the annual profit of the project. The selling price of Lithium has the largest effect on the annual revenue, and the purchasing price of raw materials is a significant variable that could change the profit by millions of dollars either way, highlighting the importance of managing material inputs.
Partners and Mentors
We would like to thank all the individuals who have provided their support and assistance throughout the Fall 2021 and Winter 2022 semesters.
Firstly, we would like to thank our supervisor, Dr. Sathish Ponnurangam, for meeting with us on a weekly basis, providing guidance and invaluable insight regarding our project design.
Likewise, we would like to thank Dr. Evar Umeozor, from the Canadian Energy Research Institute, for providing us with the opportunity to investigate the feasibility of establishing a plant in Alberta to produce lithium from oil and gas brines.
Our Photo Gallery




References
Show Us Your Canada Photo Competition. 2022. Alberta Sunrise. [online] Available at: <https://tourism.canadiangeographic.ca/entry/6808011-Alberta-Sunrise?gid=717372&offset=1&sort=title%20ASC,id%20ASC&channel=> [Accessed 4 April 2022].
Static.ags.aer.ca. 2022. [online] Available at: <https://static.ags.aer.ca/files/document/OFR/OFR_2011_10.pdf> [Accessed 4 April 2022].
German Lithium. 2022. Lithium price forecast until 2030 » German Lithium. [online] Available at: <https://germanlithium.com/language/en/lithium-price-forecast/> [Accessed 4 April 2022].
2022. [online] Available at: <https://www.thinkgeoenergy.com/preliminary-sampling-indicate-significant-lithium-grades-in-geothermal-waters-at-united-downs-project-cornwall/> [Accessed 4 April 2022].
CBC. 2022. New tech aims to extract lithium for electric car batteries from oilfield waste | CBC News. [online] Available at: <https://www.cbc.ca/news/canada/calgary/lithium-alberta-oilsands-1.5424527> [Accessed 4 April 2022].
Visual Capitalist. 2022. Charted: Lithium Production by Country (1995-2020). [online] Available at: <https://www.visualcapitalist.com/charted-lithium-production-by-country-1995-2020/> [Accessed 4 April 2022].
the Guardian. 2022. How South America’s lithium triangle is gearing up to feed our battery addiction. [online] Available at: <https://www.theguardian.com/technology/2016/mar/23/battery-lithium-south-america-chile-argentina-bolivia> [Accessed 4 April 2022].
Bmacanada.org. 2022. [online] Available at: <https://www.bmacanada.org/wp-content/uploads/AB-Li-Supply-Chain-Summary-Report_CLA_Final-1.pdf> [Accessed 4 April 2022].
Bauer, S., Chino, S., Deslandes, A., Guzmán, L. and Koop, F., 2022. Explainer: the opportunities and challenges of the lithium industry. [online] Dialogo Chino. Available at: <https://dialogochino.net/en/extractive-industries/38662-explainer-the-opportunities-and-challenges-of-the-lithium-industry/#:~:text=After%2012%2D18%20months%2C%20the,straddles%20Bolivia%2C%20Chile%20and%20Argentina.> [Accessed 4 April 2022].
Ceri.ca. 2022. [online] Available at: <https://ceri.ca/assets/files/Study_198_Full_Report.pdf> [Accessed 4 April 2022].