
The year is 2030.
You are driving west across the Prairies on the Trans-Canada Highway where a few remaining oil pump jacks nod in the heat, and then you will see it: the choppy silver waves of an immense solar array stretching in all directions. In the distance, it looks like a stranded shipping container, only a few metres in length. As you drive closer, you notice the gliding fans whirring in steel boxes. You think to yourself; this looks like a gigantic air conditioner packed in a shipping container and misplaced in the field. You are looking at a Direct Air Capture (DAC) unit, one of tens of thousands like it across the country. Together, they are trying to reverse the impact humans have had on the globe by sucking carbon dioxide directly from the atmosphere.
At DAC Engineering, we developed a detailed design for the global deployment of numerous modular DAC units. Our process has the potential to be classified as a net negative emission technology (NET) that addresses the urgent demand to decrease the anthropogenic CO2 emissions inherited from previous decades. The modular process comprises multiple separation equipment that capture and produce ~500 tCO2/year from direct air with dilute CO2 at ~400 ppm. All while being composed of only two shipping containers. In order to ensure acceleration in the innovation of our technology and potential significant reduction in costs, we incorporated free and open-source hardware (FOSH) philosophy through publishing all of the successfully completed engineering drawings, specifications, and assessments.
Join Us
Our Project

Our Team
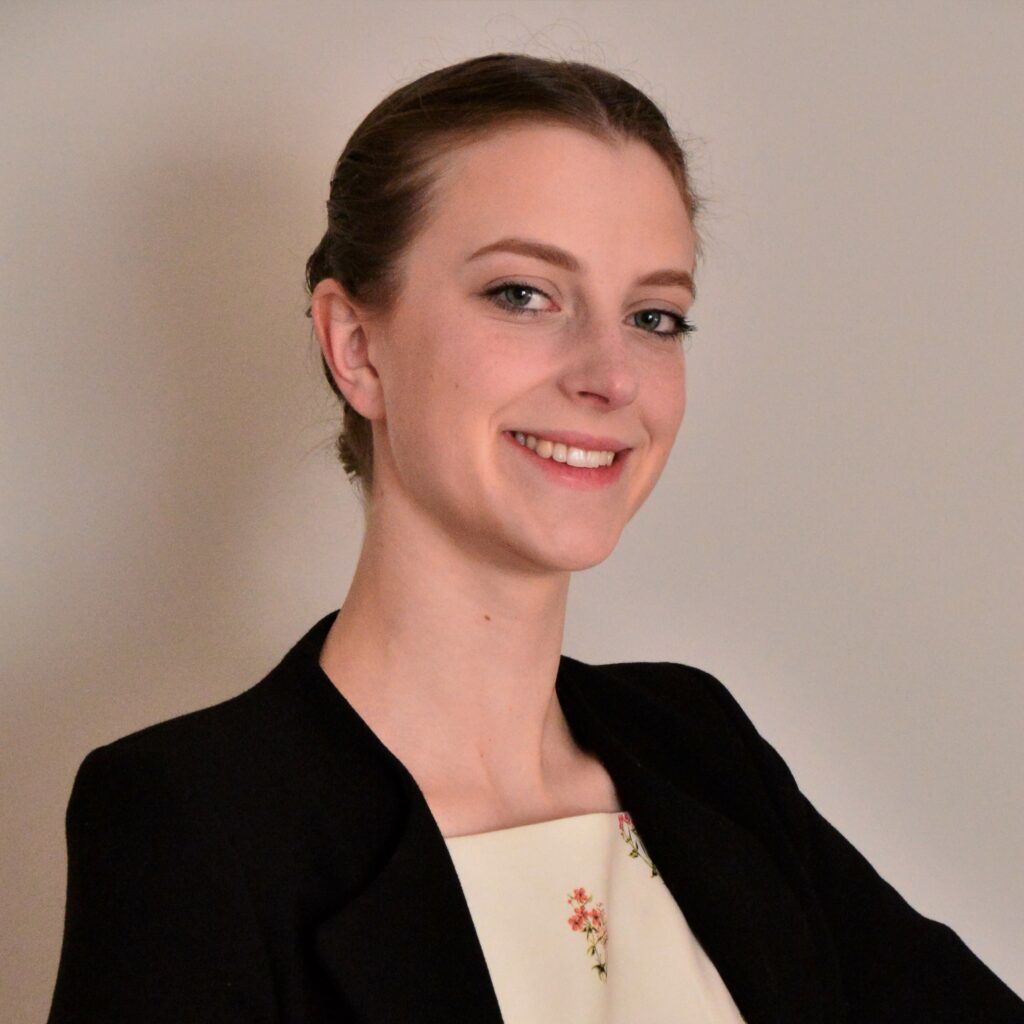
BSc Chemical Engineering
veronica.chmielarski@ucalgary.ca

BSc Chemical Engineering
dania.abukhaled1@ucalgary.ca

BSc Chemical Engineering
christy.baek1@ucalgary.ca
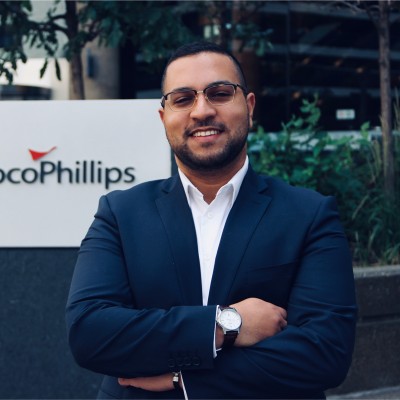
BSc Chemical Engineering
Energy and Environment Specialization
ahmad.hanafy@ucalgary.ca
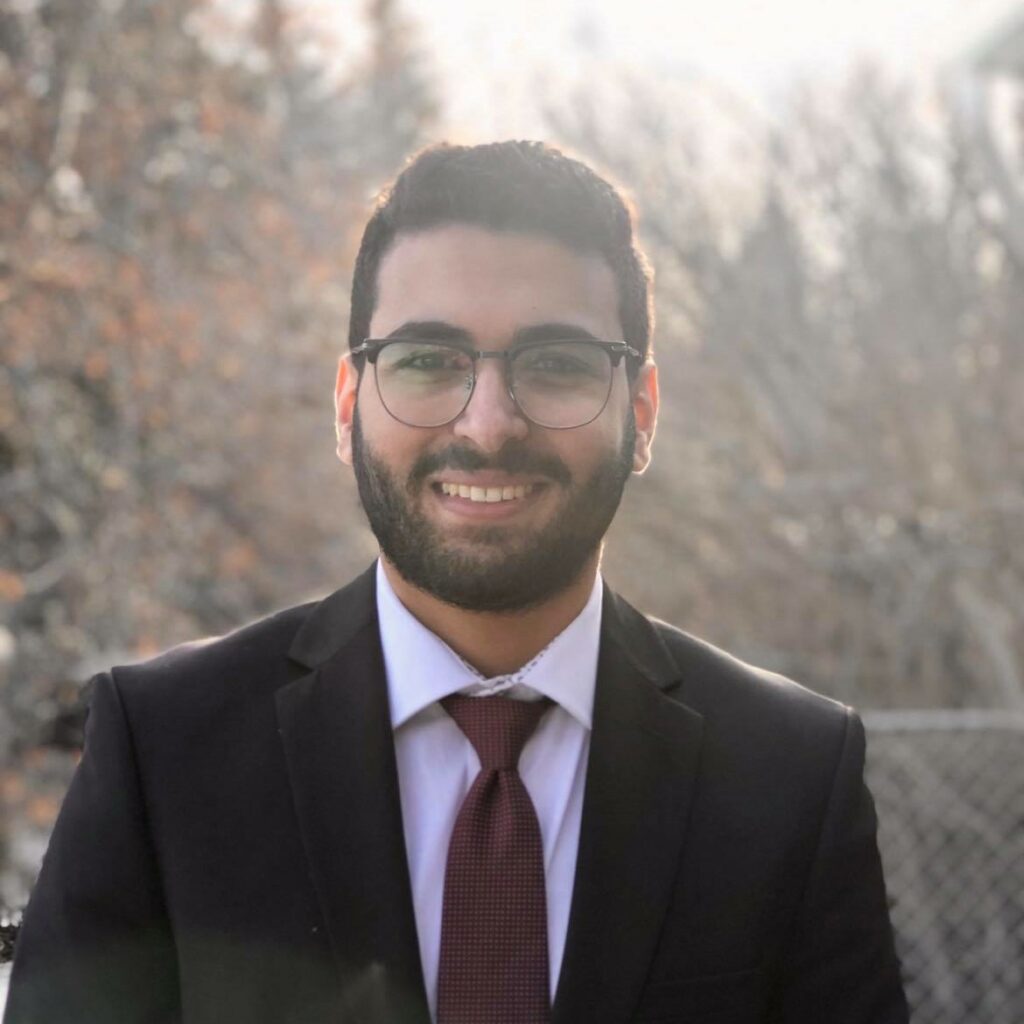
BSc Chemical Engineering
mohab.abdelaziz@ucalgary.ca
Design Details
Process Description
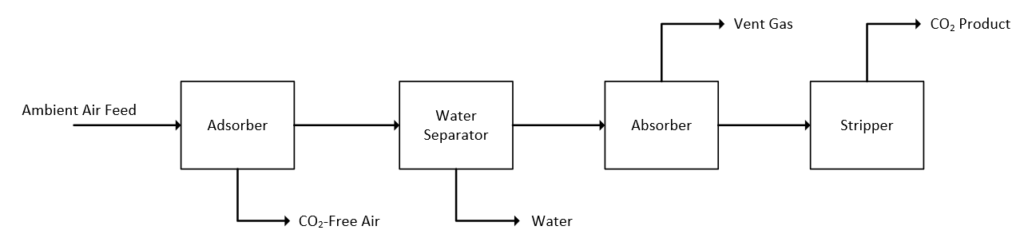
Our process begins with 10 air contacting units that implement temperature vacuum swing adsorption (TVSA) technology operated at coordinated individual times within the process cycle to adsorb CO2 from ambient air using a functionalized amine-grafted solid sorbent called Lewatit VP OC 1065. The adsorbed gas then flows to the absorption column where CO2 is captured using an amine-based solvent (MEA) in an exothermic and reversible reaction. The rich solvent exiting from the absorber is then pumped and heated before it enters the stripping column where CO2 is separated from the liquid mixture and is produced at a production rate of ~60 kgCO2/h, water with trace amounts of CO2 and MEA is recovered in the liquid phase, and the lean solvent exiting from the stripper is recycled back to the absorber.
How Our Design Addresses Practical Issues
Achieving Climate-Relevant Scale
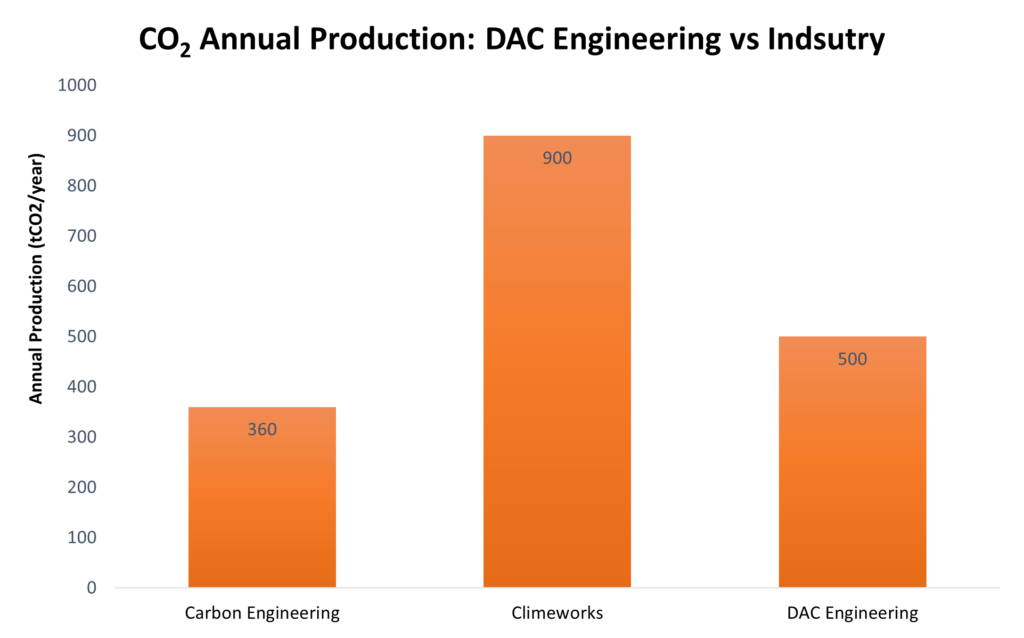
Our design addresses DAC’s biggest challenge, which is achieving climate-relevant scale. Other carbon capture storage and utilization (CCSU) technologies cannot achieve high capture capacities without significantly high cost. At DAC Engineering, we pursued mass production design basis and economies of scale by implementing tens of thousands of our modular units in order to make a dent in global emissions and achieve climate-relevant scale.
Intense Energy Requirements
Our process design tackles one of the most challenging issues the CCSU technologies encounter, which is the need of significant amount of energy (e.g. heat and electricity). Our design relies solely on electricity and utilizes only ~2670 kWh/tCO2, which is competitive when compared with other DAC technologies. Additionally, the unique design of our modular process allows for compatibility with renewable, zero-carbon energy source, which makes our process a potential net negative emission technology.

Large Scale Deployment
Current technological solutions for carbon capture already in operation are very expensive – with design and construction costs running in tens of billions of dollars – and provide minimal reduction in the overall carbon footprint. To have a meaningful impact in the trajectory of climate change, we will need to rapidly deploy tens of thousands of these multi billion dollar facilities. At DAC Engineering, we aim to deploy thousands (and millions in the future) of cost-friendly and modular DAC units, which address the current restrictions of the large scale model of CCSU technologies.
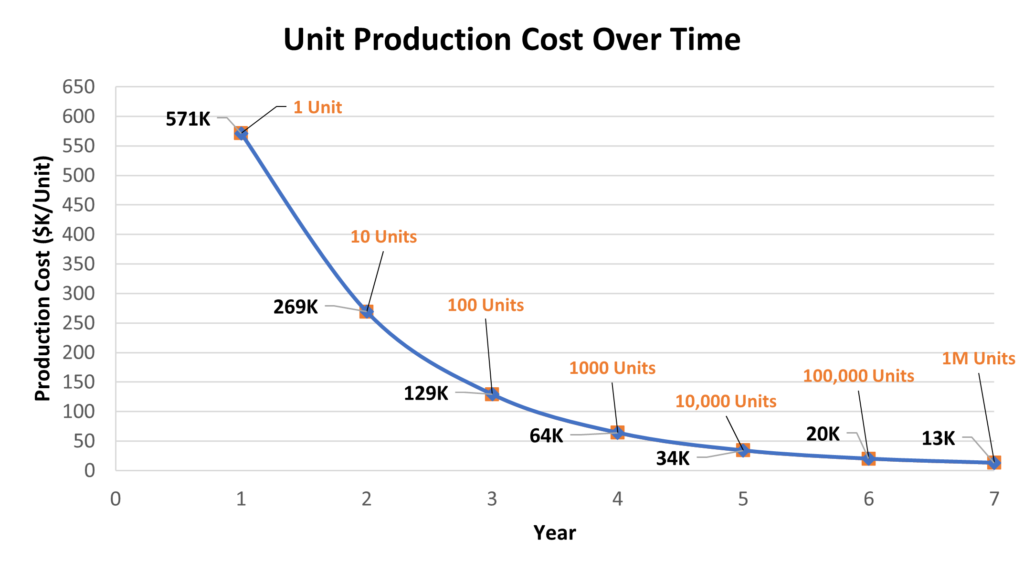
DAC Engineering aims to produce 10X more units every year
Technological Barriers
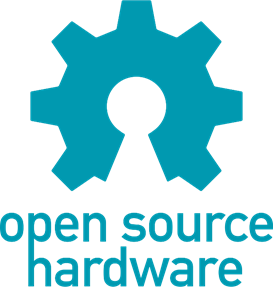
As technologies become more interdependent and innovation relies more and more on fragmented proprietary knowledge, concerns are emerging about the innovation becoming more difficult and the commercialization of technologies being held backed by patents.
The beauty of open source is that it is a huge ecosystem of innovators who are no longer competing for scarce resources but rather sharing knowledge with others to create new resources and opportunities for others to benefit from these resources. Therefore, our design encompasses several aspects of the free and open-source hardware methodologies such as global applicability, availability of materials, and more.
What Makes Our Design Innovative
Geographically Flexible
Most carbon capture focuses on cleaning emissions at the source: scrubbers and filters on smokestacks that prevent harmful gases reaching the atmosphere. But this is impractical for small, numerous point sources like the planet’s billion or so automobiles. Nor can it address CO2 that is already in the air. That’s where DAC comes in. DAC’s ability to capture CO2 directly from air is one of its biggest drivers as it can be placed anywhere in the world and will be effective. Our design in particular would be considered an extremely flexible unit as it is placed in a shipping container, which means that it can be shipped and deployed anywhere in the world offering unparalleled geographical flexibility.
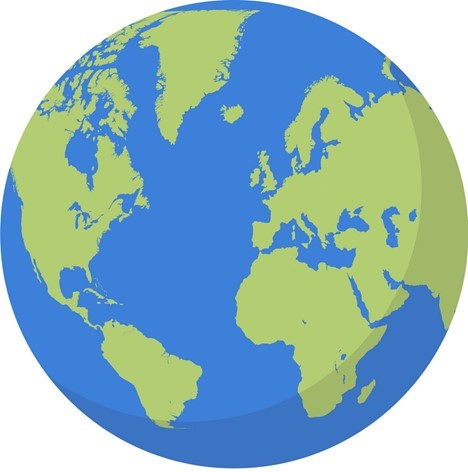
Utilizing Air for Efficient Heat Exchange
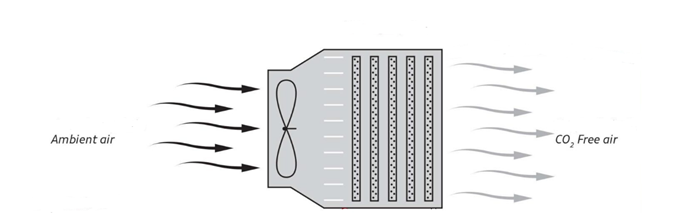
One of the innovative aspects of our design is that we utilize the air flow as one of our main heat exchange mechanisms. Our process intakes a massive amount of air that goes through the air contactors and as carbon-free air exits these contactors, we utilize the existing air stream to operate our three air-cooled heat exchangers, saving close to $700,000 in energy costs.
Diversity of Utilization
Close to 230 million tons of CO2 is used every year, mainly in the fertilizer industry and in the oil and gas industry for enhanced oil recovery (EOR) applications. Placing our unit around chemical plants will enable the production companies to use the captured CO2 in their oil recovery process, exhibiting one of the best examples of circular economy.

Furthermore, CO2 is widely used in commercial applications including food and beverage production and building materials. Our sponsor, Carbon Upcycling Technologies has a consumer goods eCommerce site called Expedition Air, which utilizes their carbon do create products that incorporate CO2. Making low-carbon materials accessible to all supply chains, which allows and empowers consumers to use their buying power to enforce the need for products made from CO2 help rather than hinder our planet.

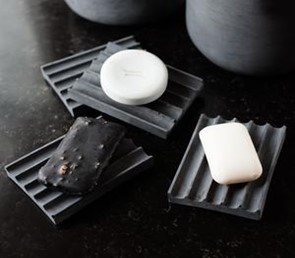
NET Potential

Since our process only utilizes electricity and is at a relatively small scale, these containers can be strategically placed around solar or wind farms in order to harness their energy. Using renewable, emission-free energy sources to capture CO2 will guarantee that our process becomes a net negative emission technology.
What Makes Our Design Solution Effective
Designed for Varying Conditions
Since our process intakes air as an input feed from the atmosphere, variation in the feed parameters is expected. Our DAC unit incorporates the fluctuations and variation aspects of temperature, pressure, moisture content, and CO2 and water concentrations in air. In order to ensure a year-round successful operation, our process has been designed to encompass two average seasonal cases (summer and winter) for our pilot location basis, Calgary, Alberta. To ensure an effective design, we utilized the two seasonal cases results to size our DAC unit. Additionally, we have completed sensitivity analysis on extreme weather conditions to study how our process would respond to these fluctuating weather conditions.
Tangible Production Rate with High Purity
Our units are capable of producing close to 500 tCO2/year, which can be utilized in various ways as mentioned. However, what makes our design effective is the high degree of purity that CO2 is produced at. With a purity greater than 96%, our product is suitable for all various inputs for downstream processes such as EOR.
Economies of Mass Production
One of the main issues that is faced by industry is the requirement of a large capital expenditure to set up new carbon capture plants. For instance, in November 2015, Shell and CNRL began operating Quest, a carbon capture project from point source emissions that costs more than $1.35 billion CAD with more than 64% of that capital cost coming from the government and subsequently taxpayers. High initial cost and feasibility associated with these projects may hinder market growth and is perhaps one of the biggest restraining factors to CCSU.
Rejecting the big-is-better ground rule of industrial production has profound implications for production strategy decisions in the future. Leaders must now consider some of the advantages of small scale models such as reduced risk, financial flexibility, and geographic compatibility as they plan the future infrastructure of their companies.
How We Validated Our Design Solution
Adsorbers
Since adsorption is a batch process, modelling of the adsorber unit mainly surrounded modelling the adsorption mechanisms. The CO2 capture mechanism surrounding the adsorber has been mathematically approximated using the Toth equilibrium and kinetic CO2 adsorption models, which have been shown to have a standard deviation (SD) of 11% in dry conditions and 16.4% in wet conditions in comparison with the experimental results.
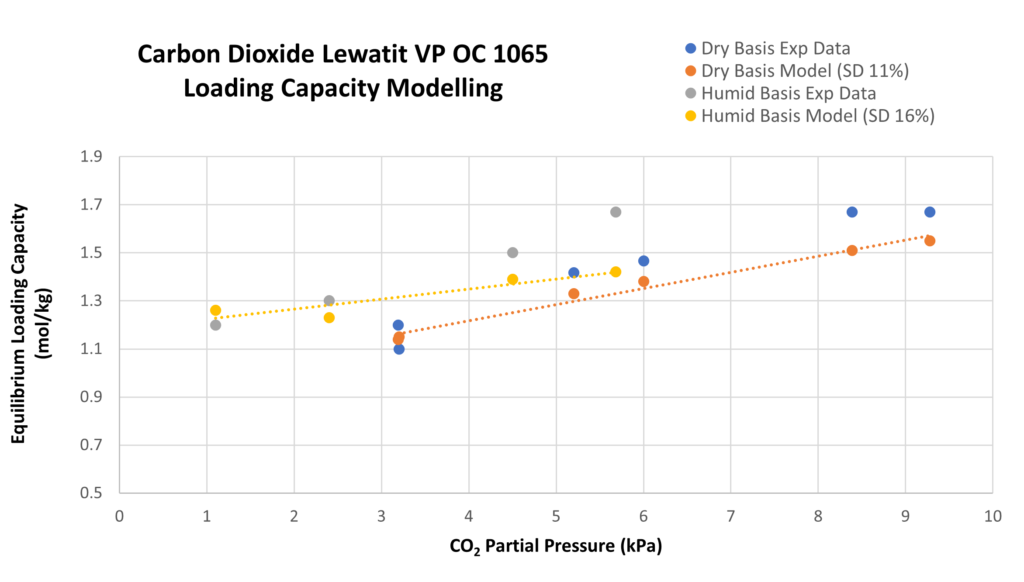
Other aspects of our process design were validated by using Aspen Plus alongside rigorous literature analysis.
Absorber
The absorption column simulation in Aspen Plus was validated against a pilot plant column operating at similar conditions. The Electrolyte-NRTL thermodynamic model was used to predict the vapour-liquid equilibrium of the system. This is due to the model’s ability to take into consideration the non-ideality of the liquid phase due to the presence of ions and based on literature, this is the most suitable and accurate model. The liquid temperature was compared to the benchmark data and as seen in the figure below, was successfully matched.
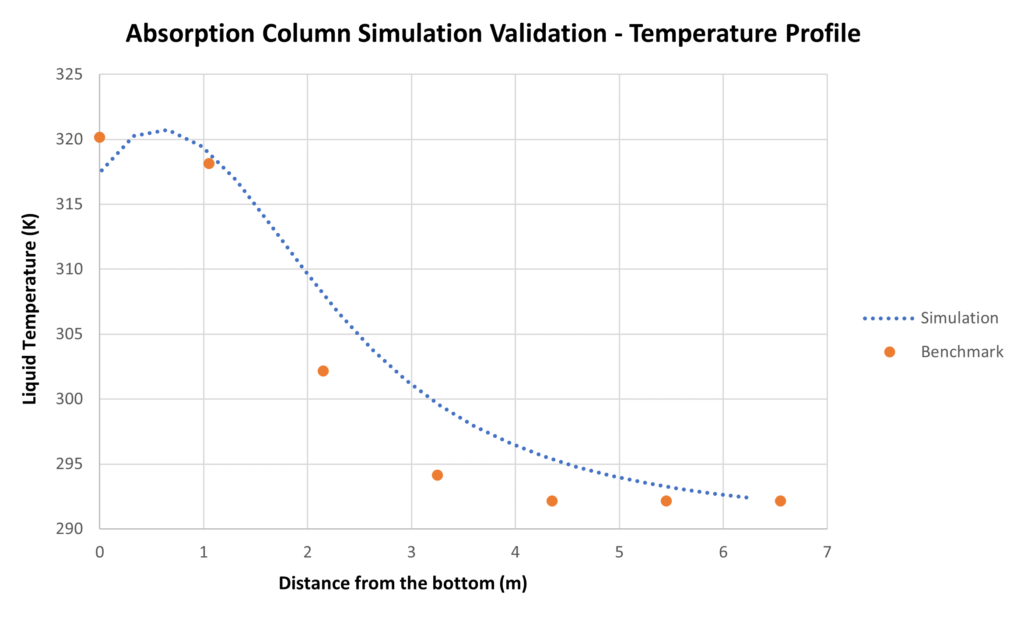
Stripper
Additionally, the stripping column’s specific reboiler duty was compared to two distinct literature values and as seen in the figure below, was determined to be within the acceptable range.
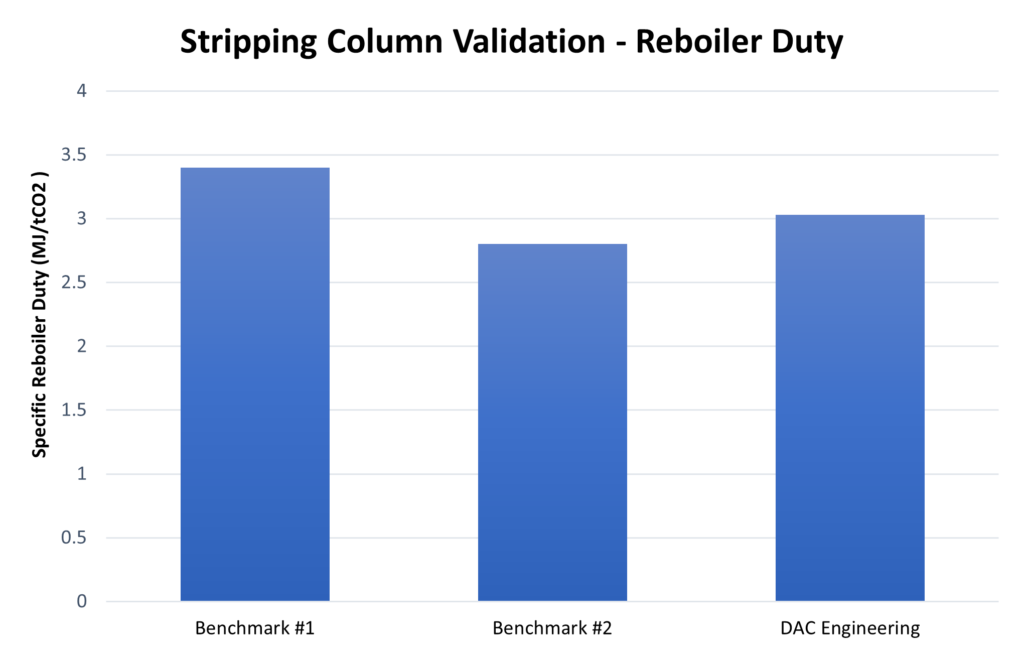
Heat Exchangers
The air-cooled heat exchangers and cross heat exchanger were modelled and simulated by using Aspen EDR and further validated with hand calculations regarding heat duty. Also, the temperatures were checked by using Aspen Energy Analyzer to ensure that there are no unsatisfied or unfeasible streams.
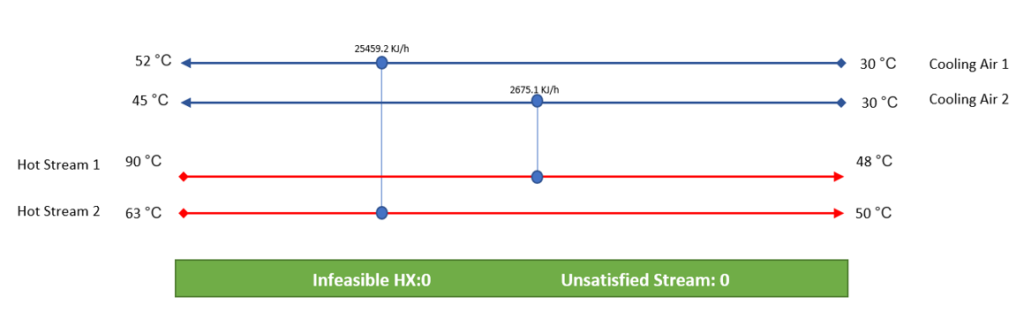
Feasibility of Our Design Solution
Energy Feasibility
Energy consumption comparison with some industrial processes supported the feasibility of our design that it is in the acceptable energy consumption range.

Economic Feasibility
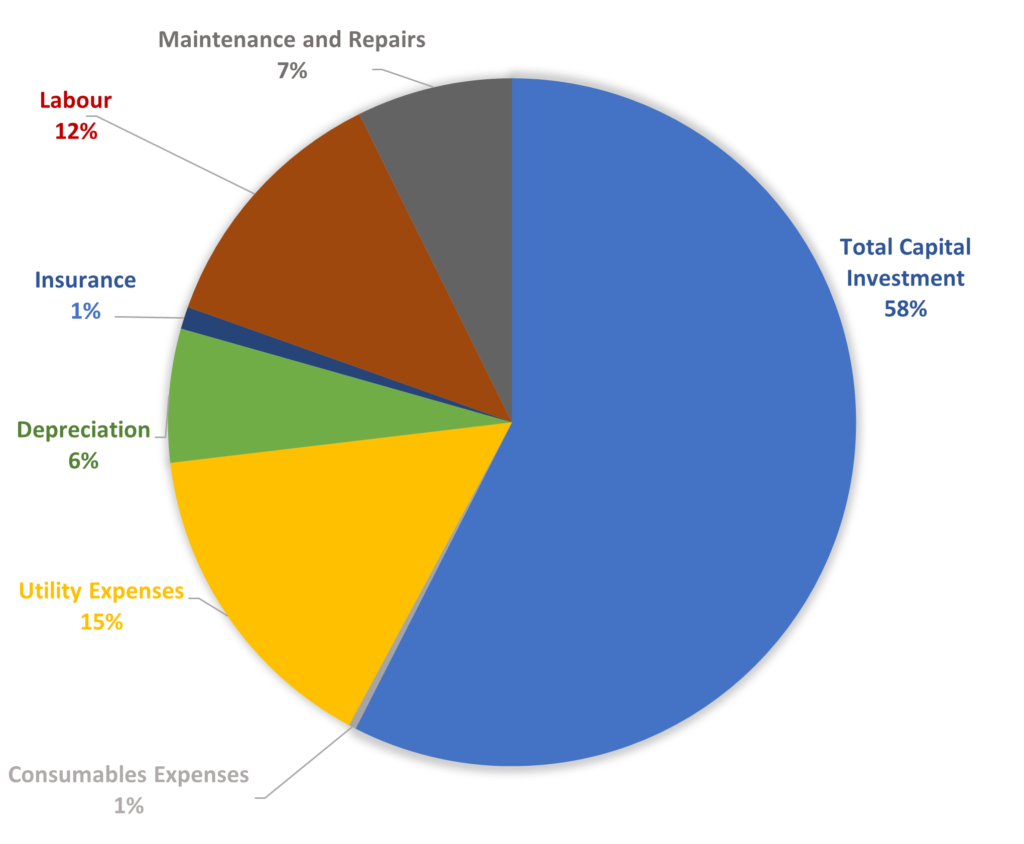
Our business venture successfully demonstrated the economic feasibility for DAC Engineering and our Client:
- Developed a financial analysis of our 2-year pilot DAC unit and the total investment (capital and operation) was identified to be $960,000
- Conducted economies of large mass production strategy to scale up output and unit productions. The results demonstrated our capacity to target a steady production of 10,000 units per year at a unit production cost of $36,000/unit
- Developed a financial analysis that identified our Client payback period to be 5 years at a unit selling price of $150,000, carbon selling price of $0.24/kg, and NPV of $85,000. The results indicated that DAC Engineering net cash flow is $92,000 with NPV of $74,000
Acknowledgements
DAC Engineering team would like to specially thank our supervising professor, Dr. Hector De la Hoz Siegler for his continuous guidance and support. We consider ourselves privileged to have his supervision.
We would also like to thank our sponsor, Carbon Upcycling Technologies for their support, sponsorship, and shared vision.

Photo Gallery
The world’s first commercial carbon capture plant opens in Switzerland, 2017.
https://www.dezeen.com/2017/06/06/world-first-commercial-carbon-capture-plant-switzerland-pollution-technology-news/Direct Air Capture Technology, 2021. Carbon Engineering.
https://carbonengineering.com/our-technology/
3D Model Angle 1 3D Model Angle 2