Project Category: Mechanical
Consumer Level Wind Turbine
About our project…
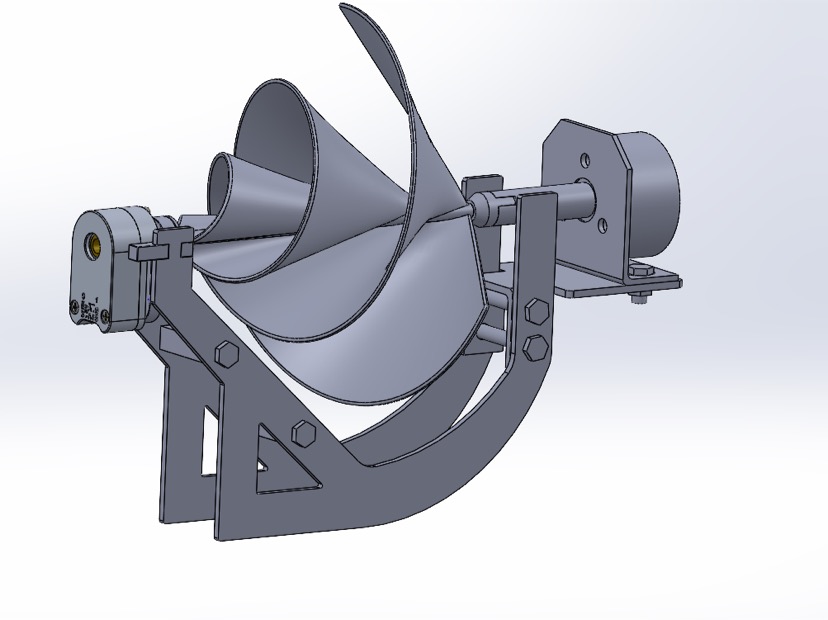
Our project centered around the design and validation of consumer level wind turbines utilizing wind tunnel testing of scale models. We investigated the advertised performance of a currently available turbine on behalf of a Calgary construction developer. This includes both the power generation and thrust loading on an Archimedes wind turbine.
We created our own modified Savonius turbine design. We applied the same verification tests used for the Archimedes to our designs. It has comparable performance to the Archimedes while being easier to manufacture.
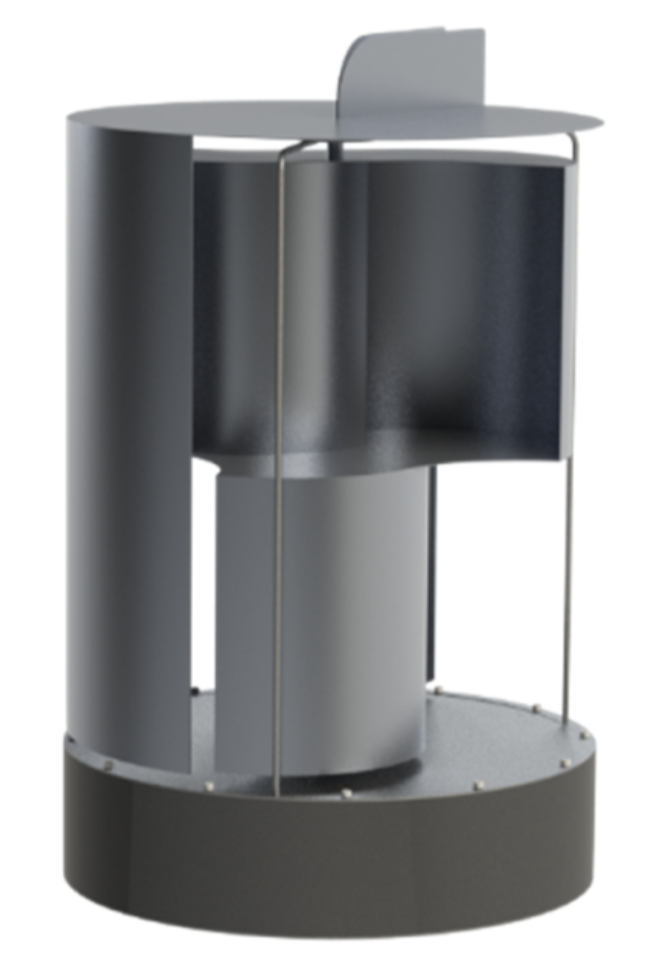
How does our design address practical issues?
Growing industry and population worldwide has caused a significant rise in energy demand over the last decades. To meet this demand, energy production has also increased. Small scale energy generation, or microgeneration, is becoming an increasingly feasible method of supplementing this rise in demand and providing reliable energy for homes and businesses.
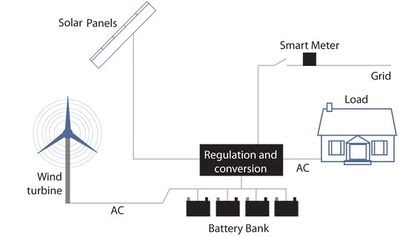
What makes our design innovative?
Few independent studies have been completed on the Archimedes wind turbine design. Our work looked to evaluate the performance of the turbine for a Calgary building developer. Additionally, our thrust analysis has led to the first reported thrust coefficient for the Archimedes wind turbine.
Our modified Savonius wind turbine implements a cover design that provides increased performance over the traditional arrangement while maintaining a simple and compact model. For more information on our final design click here.
What makes our design solution effective?
We were able to complete a full performance and thrust loading analysis of the Archimedes turbine using our scale model. This allowed us to give our sponsor critical information regarding their decision to become a distributor.
Our design is simple, easy to manufacture, and provides good power performance at low wind speeds. The overall performance is comparable to that of the Archimedes turbine. For more information on the comparison click here.
How we validated our design solution?
The project was given the opportunity to utilize the wind tunnel facilities at the Laboratory for Turbulence Research in Aerodynamics and flow Control (LTRAC) at the University of Calgary. Our prototypes were created for use in the wind tunnel, with their scale and mounting reflecting this. Scale model testing formed the basis for our design iterations and verification of overall performance. For more information on click on Methods and Results.
Feasibility of our design solution?
Our turbine consists of a modular design with a majority of the components able to be cut from sheet metal and welded in place. The modules and components were designed in such a way that no complex tools or fixtures are required for assembly. All of this allows for ease of manufacturing, assembly, and maintenance. This makes the turbine more accessible to consumers, both in terms of cost and operation. For more information on our final design click here.
Details about our Project
Prototype Design and Manufacturing
Archimedes Turbine Prototype
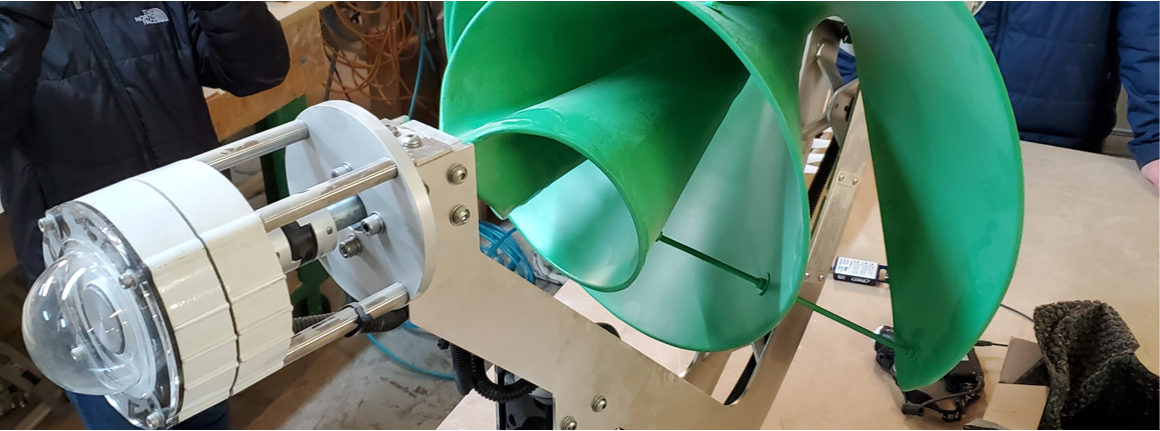
The Archimedes
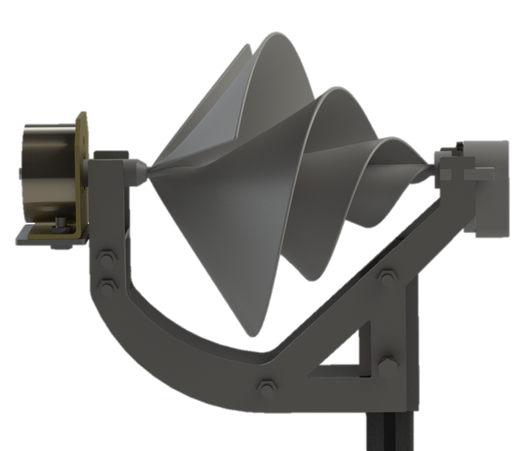
A 1:4 scale model of the Archimedes was designed using Solidworks based on the dimensions of the testing equipment. The model was then subject to a few minor changes to fit within the wind tunnel.
We manufactured our prototype using a combination of 3D printing, laser cutting, epoxy, and welding.
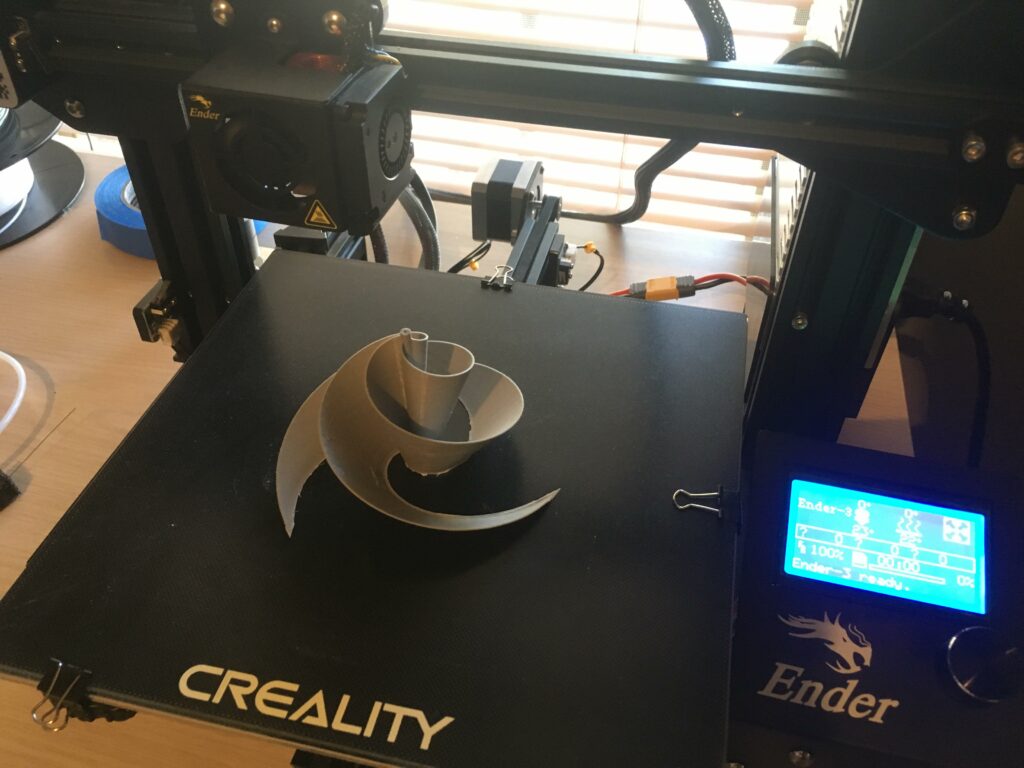
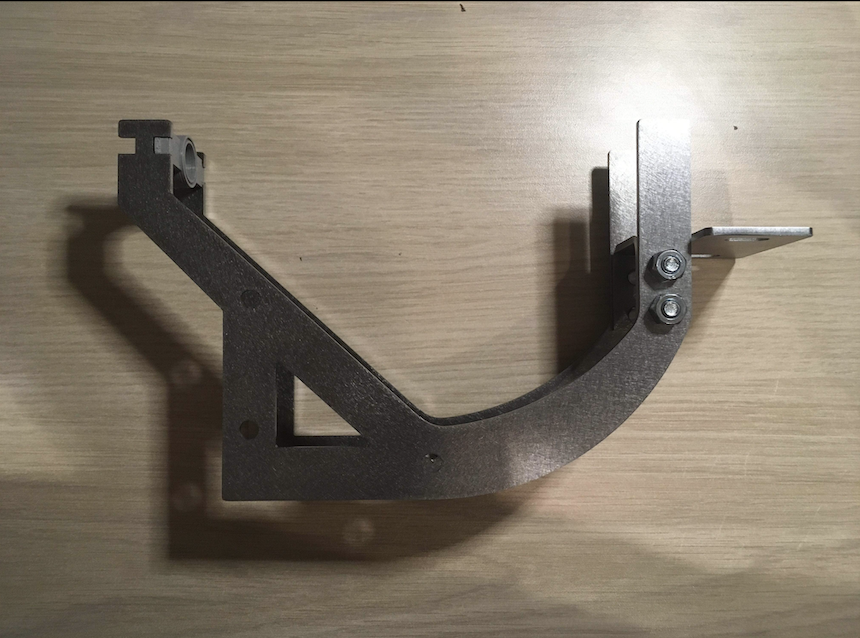
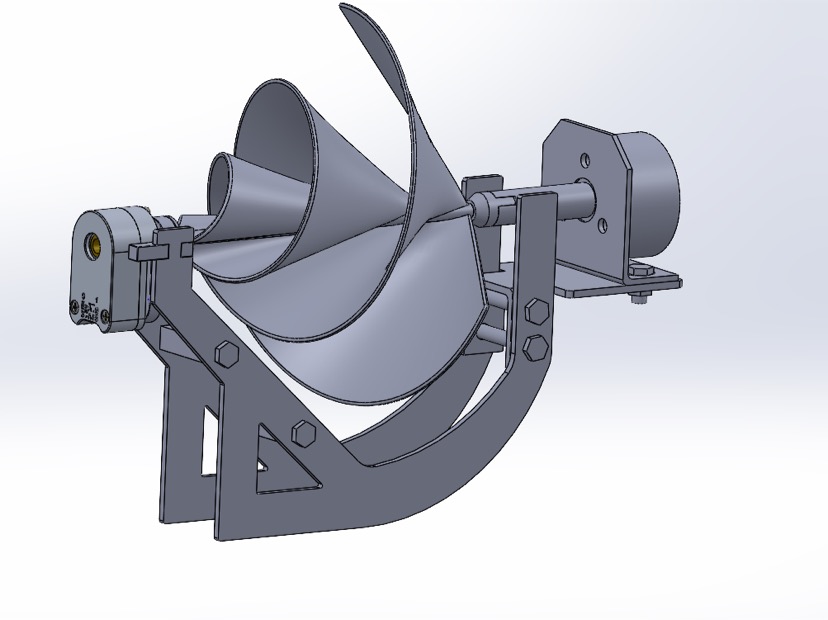
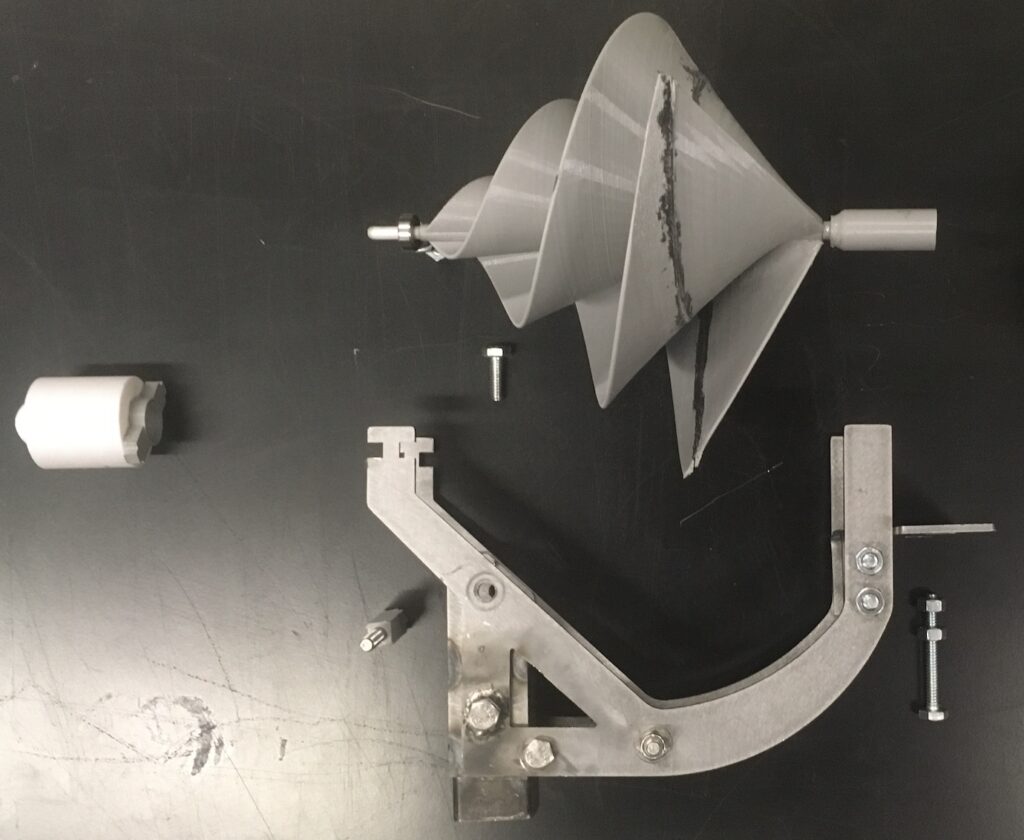
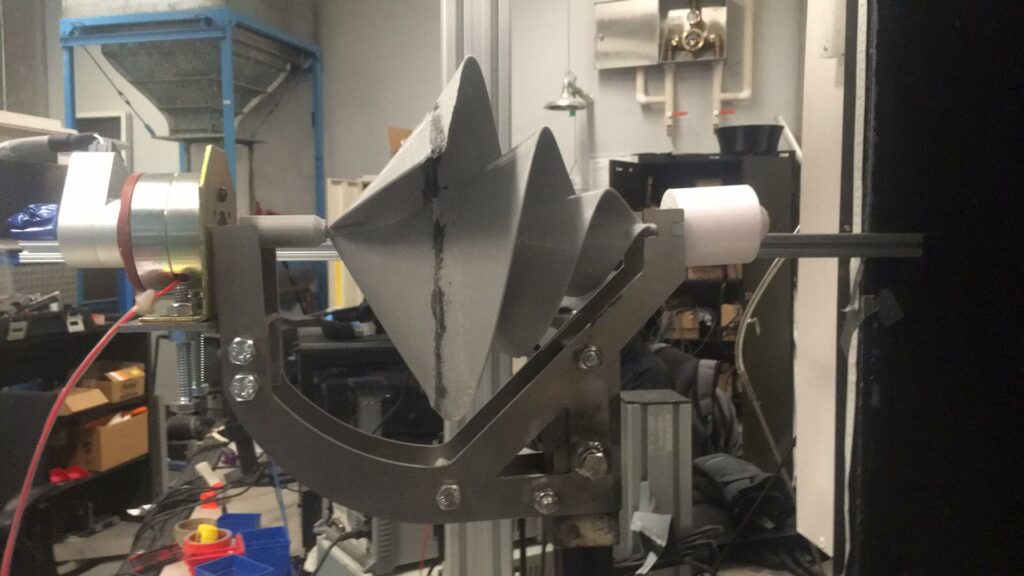
Vertical-Axis Wind Turbine Prototype

Vertical-Axis
Wind Turbine
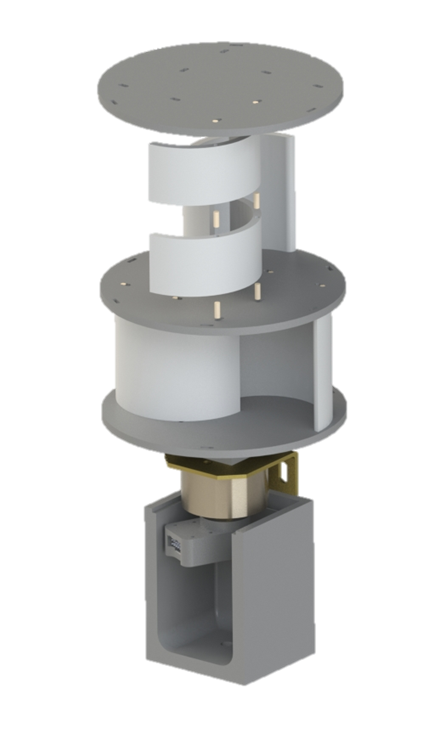
We incorporated modular design philosophies into our vertical-axis turbine prototypes to individually examine various parameters. This was so we could test a combination of parameters to achieve a performance comparable to the Archimedes.
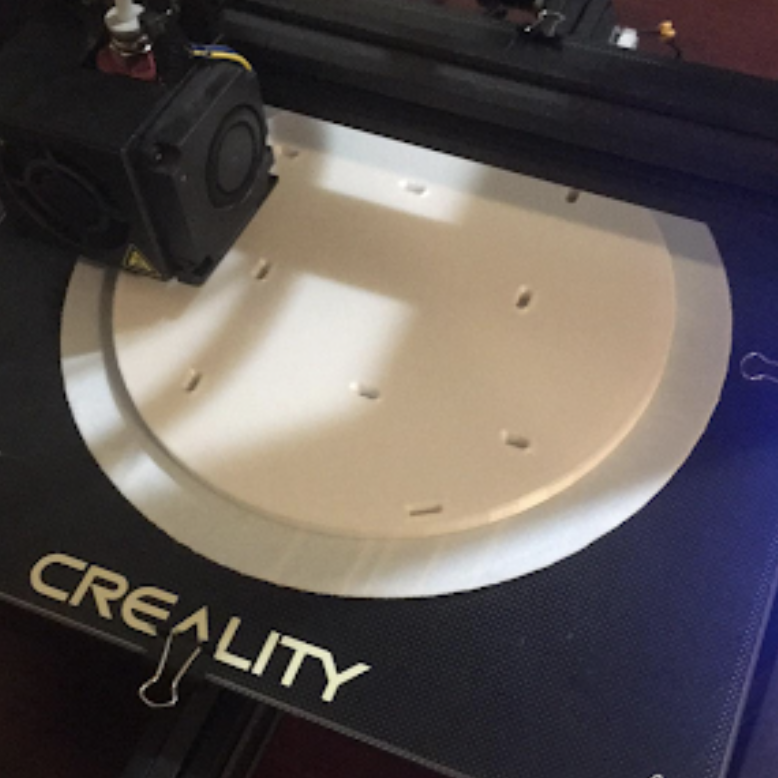
For our prototype, all major parts were 3D printed using a personal 3D printer and the University of Calgary Maker Multiplex.
We investigated the effects of using a cover to shield the returning blade of the Savonius Turbine. This was found to reducing the cut-in speed of the turbine. Additionally, it increased the rotational speed (RPM) and performance of the turbine.
Design Verification
To verify our design in comparison to the archimedes, we conducted wind tunnel testing within the LTRAC facility of the Energy, Environment and Experiential Learning (EEEL) building at the University of Calgary Campus.
Performance Metrics
Tip Speed Ratio (TSR)
The ratio between the wind speed and the blade tip speed. In other words, a larger radius or faster spinning blade will have a larger TSR.
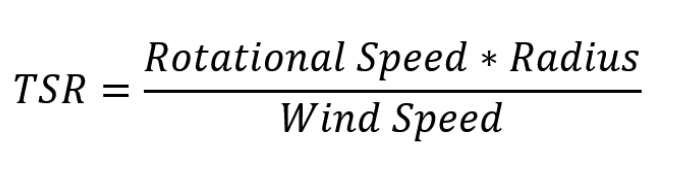
Coefficient of Power (Cp)
The fraction of the available energy in the wind that a turbine can harvest. The maximum theoretical Cp for a turbine in realistic settings is 0.59, referred to as the Betz limit.
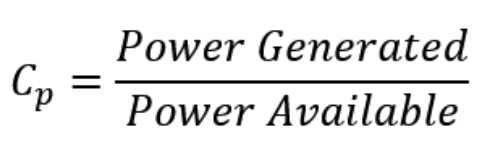
Coefficient of Thrust (Ct)
The Thrust Coefficient helps represent the expected loading on the turbine. This is essential for determining the structural requirements for mounting the turbine.

Data Collection and Testing
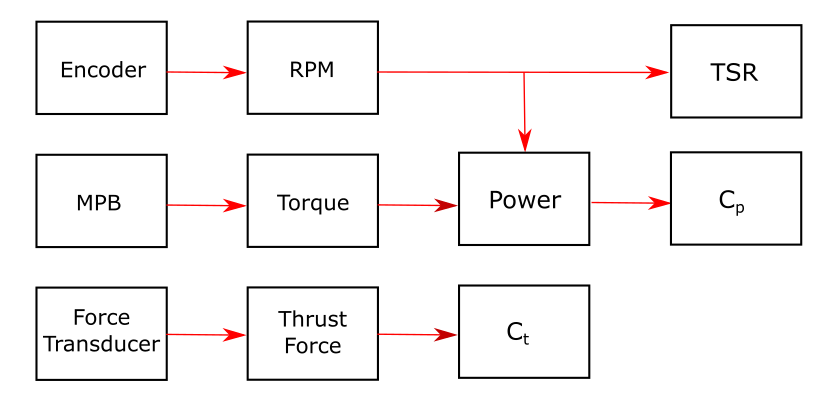
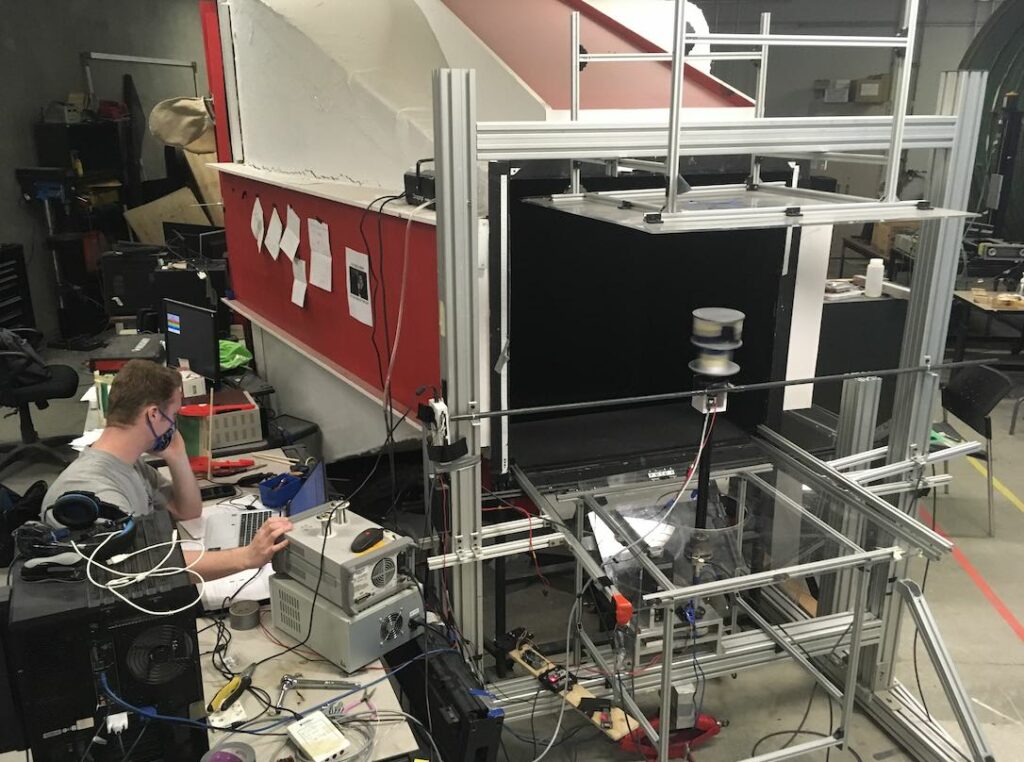
Rotational Speed (RPM)
The rotational speed of the turbine is measured using a rotary encoder connected to an NI MyDAQ module. It was mounted to the shaft of the turbine in line with the MPB, allowing us to capture the RPM output of the turbine.
Torque
The torque produced by the turbine was measured by simulating the load by a generator. This was through utilizing a magnetic particle brake (MPB). We completed a calibration of the MPB to ensure we could correlate the supplied voltage with the torque produced.
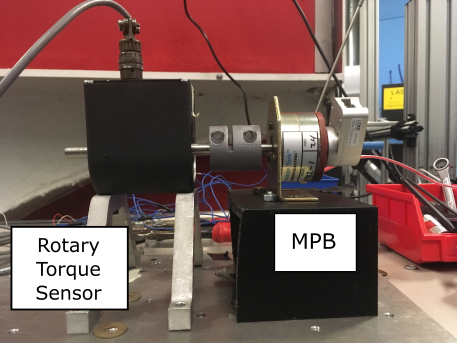

Thrust
A 6-Axis ATI Force-Torque transducer was utilized to collect the thrust force in the wind direction. It was sampled at 100Hz and calibrated independently from this project by the manufacturer. For our experiments, the thrust force is measured as the force in the axis of the wind direction (x-axis of the transducer). The transducer was supplied with an input voltage of 18V and the DAQ used was a NI6212 USB module.
Experimental Results
See below the results for the Archimedes or the Savonius Models.
The Archimedes Turbine
Using RPM and torque data collected from multiple tests a relationship between the power coefficient and tip speed ratio was developed. Our results show the Archimedes turbine reaching a maximum power coefficient of approximately 0.27 occurring at a tip speed ratio of 2. This information is useful when designing a controller for the turbine. An ideal controller utilizes a braking system to ensure that the turbine remains at a tip speed ratio of 2 regardless of the wind speed, providing the largest power output possible for each wind speed.
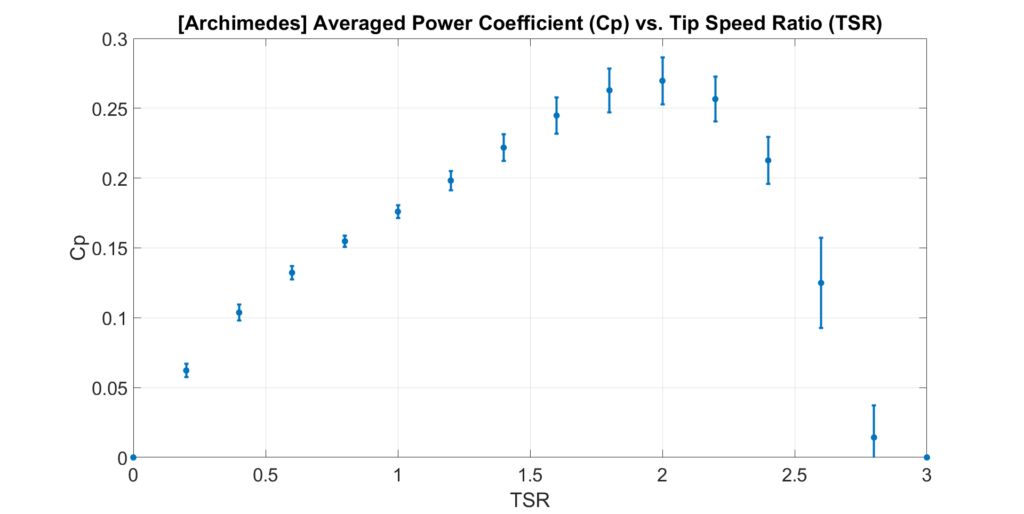
Utilizing the maximum power coefficient of 0.27 our experimental results could be extrapolated to investigate the performance of a larger, more realistic Archimedes turbine. The power curve for a 0.75m diameter Archimedes turbine was calculated, showing the power output across a wide range of wind speeds. To obtain a more realistic result an estimated energy efficiency of 75% was also applied to account for energy losses due to the controller and generator required to operate the turbine.
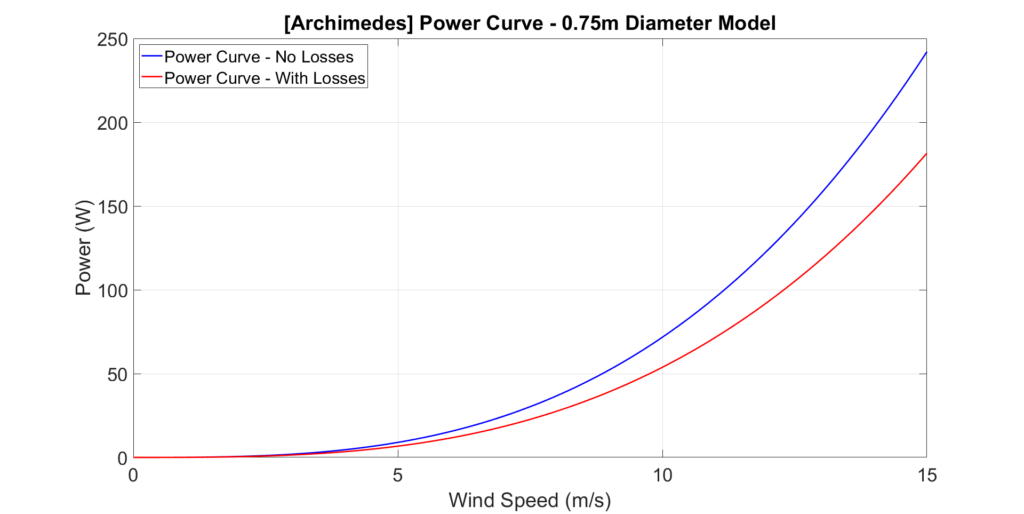
Along with the power performance, the thrust characteristics of the turbine were also analyzed. Using thrust data collected at multiple wind speeds the thrust coefficient of the Archimedes turbine was calculated to be 1.09. Like the power coefficient, the thrust coefficient was then used to estimate the expected thrust forces for a 0.75m diameter Archimedes turbine. This thrust curve provides useful information when analyzing the structural requirements for mounting the turbine.
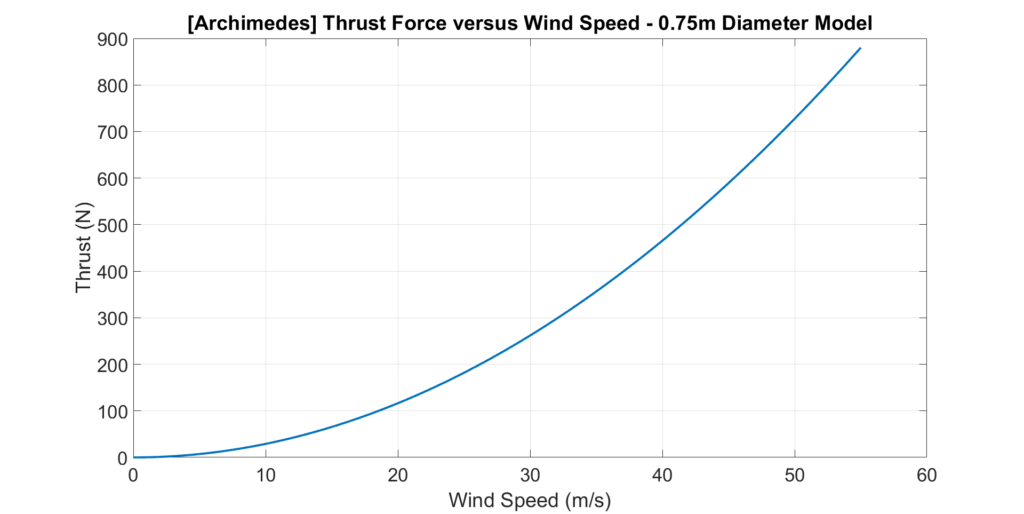
The Savonius Turbine
Several potential overlap ratios were tested for the Savonius turbine blades. The power output with each ratio was calculated to determine which provided the best performance. Through our experimental testing the best overlap ratio was 0.25.
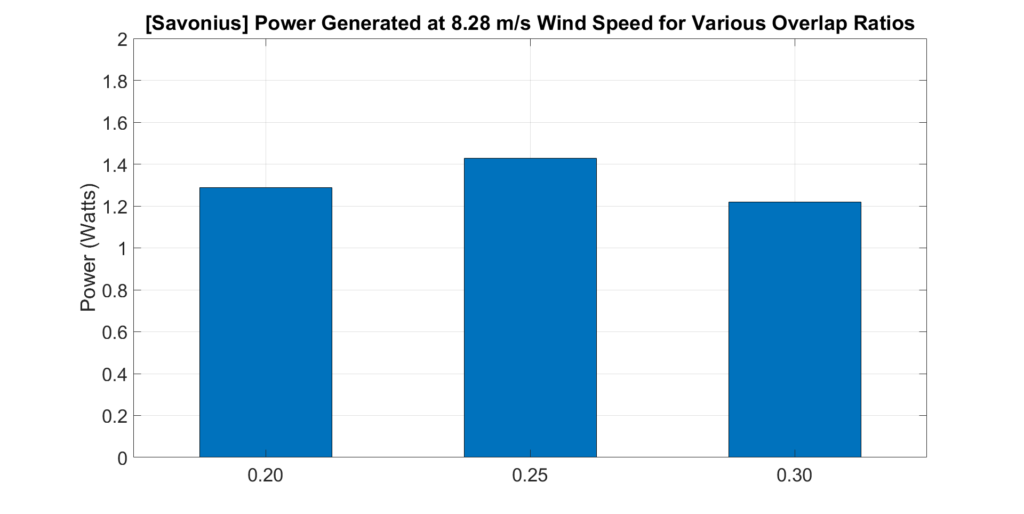
Several tests were done without the cover design to determine a baseline power output for the Savonius test model. The cover was then added and the same tests were performed. The addition of the cover provided a 77% increase in output power at low speeds, decreasing to 3% at higher wind speeds. Overall, the addition of the cover proved successful in reducing the starting speed of the Savonius testing model as well as its power production.
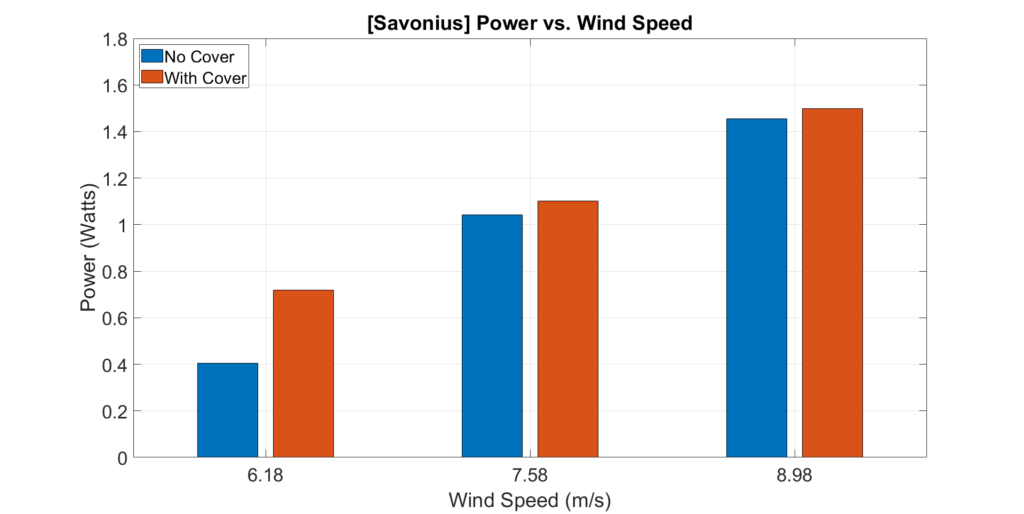
Like the Archimedes turbine, a relationship between the power coefficient and tip speed ratio was developed. Testing results showed that the Savonius power coefficient relied heavily on wind speed, decreasing as the wind speed was increased. Using this information a power curve for a 0.75m diameter Savonius turbine was calculated. Again, a 75% energy efficiency was applied to account for losses due to both the controller and generator required.
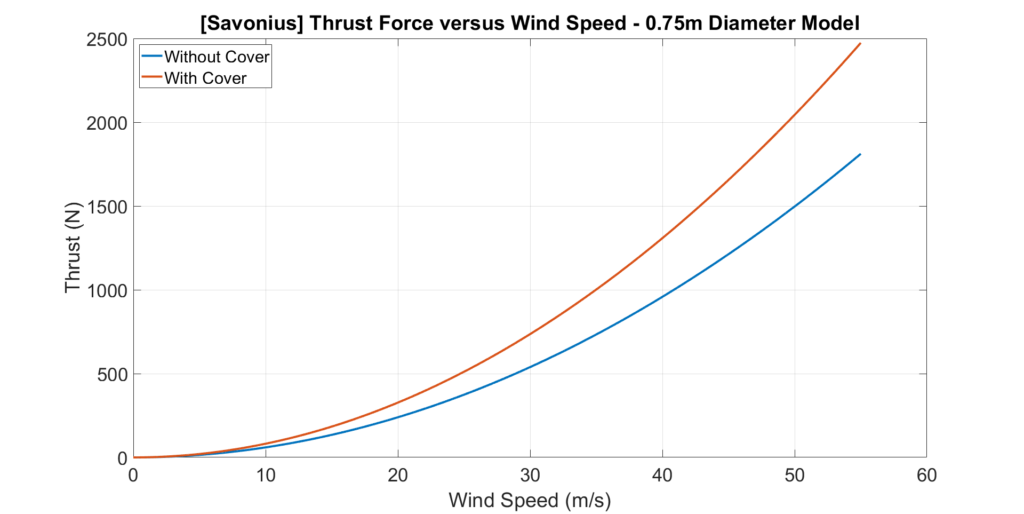
The thrust coefficient for the Savonius turbine was calculated both with and without the cover. Without the cover, the thrust coefficient was 2.02 increasing to 2.76 when the cover was added. These values were then used to develop thrust curves for the 0.75m diameter Savonius turbine with and without the cover.
Comparison
Our results for the Archimedes turbine were compared to the power performance provided by a manufacturer of the Archimedes turbine. Our model shows slightly lower power output than that advertised by the manufacturer.
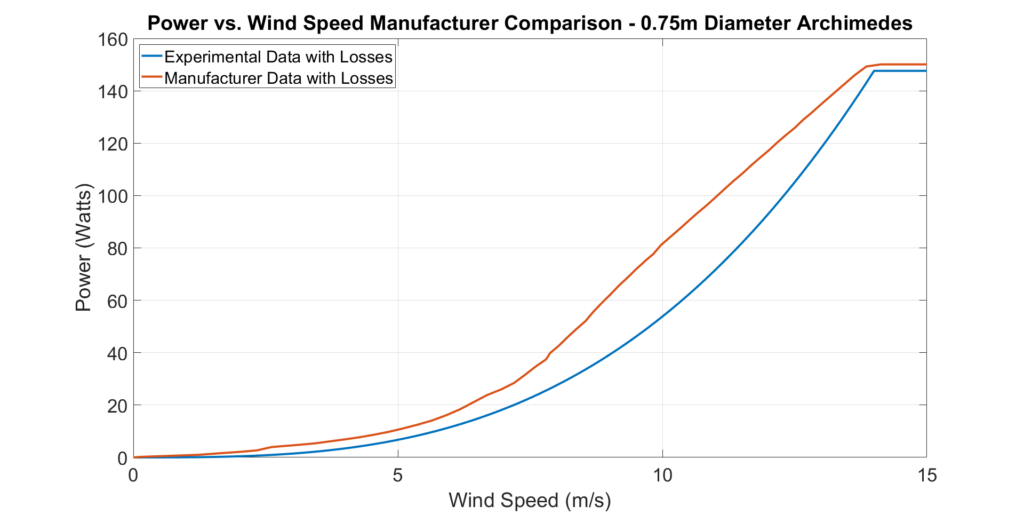
Additional comparisons were done between the Archimedes and Savonius turbine models. Using annual wind data collected from the Calgary International Airport, the annual power production for each design was calculated.
Based on research done over the last decade, the average household in Alberta consumes approximately 7,200 kWh of electrical power per year. One 0.75m diameter Archimedes turbine can provide 3% of that annual power. One Savonius turbine provides approximately half that of the Archimedes, or 1.5% of the annual power required. It is important to note that the goal of these designs is not to power an entire household, but to provide a form of microgeneration that supplements other power production methods.
Turbine Model | Annual Energy Production (kWh) |
---|---|
Archimedes | 227.8 |
Savonius | 111.5 |
Final Design
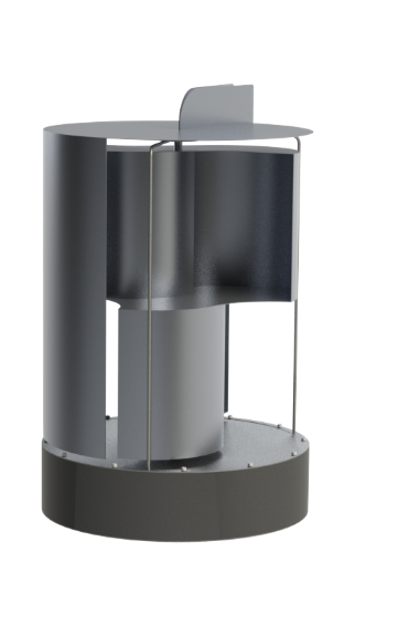
Final Design
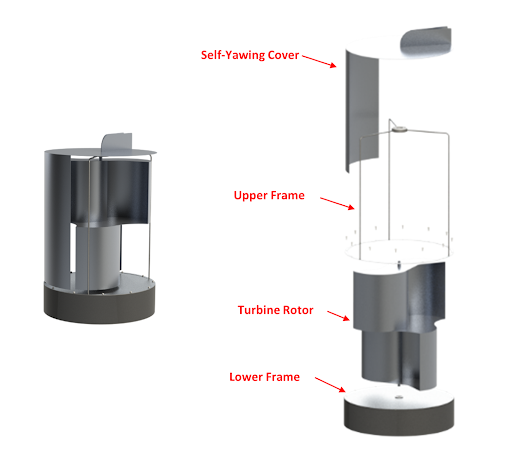
The role of the cover is to improve the performance of the turbine by shielding the convex faces of the returning blades. The cover consists of three main components: The top plate, shroud, and the wind vane. The top plate acts as a mounting point for the other components whilst also connecting to a ball bearing on the upper frame, allowing the cover to rotate freely. The shroud serves to shield the returning blades of the turbine from oncoming wind. All the components are made from 14-gauge 6061 aluminum sheet, allowing them to be cut and rolled to form along with the rotor components. They are joined in their respective positions with intermittent GTAW welds. Again, the use of 6061 aluminum results in a total mass of 5.6kg, allowing it to rotate with ease to align with the direction of the wind.
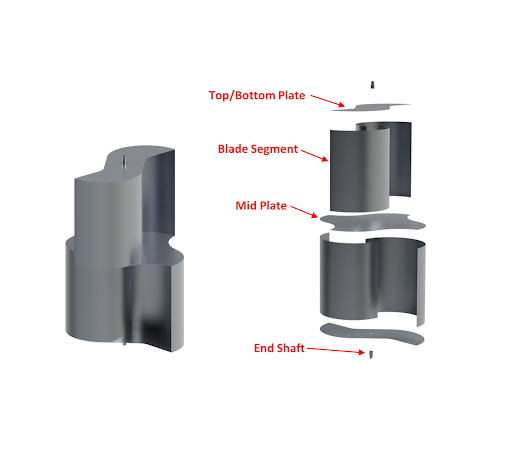
The turbine rotor is the main functional component of the turbine. It is responsible for capturing the kinetic energy of the wind and using it to power the generator. Based on the results of our wind tunnel testing, a combination of design parameters was chosen for best performance. It features a stacked blade design in which the blade stacks are rotated 90 degrees relative to each other. The aspect ratio of the turbine is 2 and the overlap ratio is 0.25.
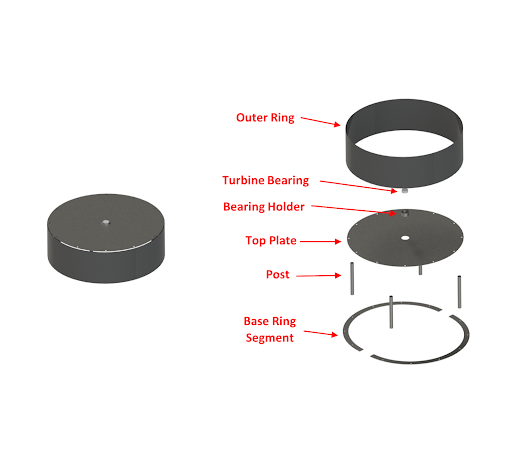
The lower frame is the base of the unit. It contains the lower bearing which supports the turbine and provides a mounting point for the upper frame assembly. It is also the part of the turbine which is mounted where the unit is installed. Inside of the lower frame, there is ample room and mounting points for the generator and control electronics that would be fitted to the turbine.
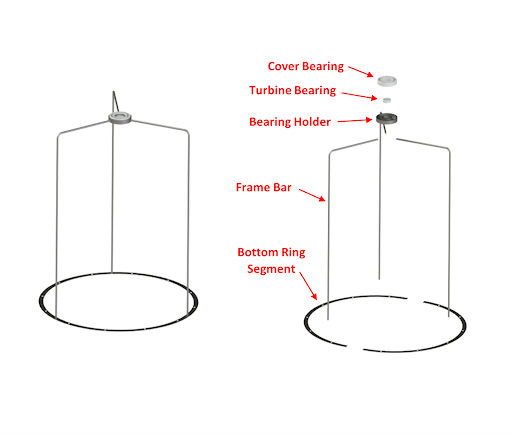
The purpose of the upper frame is to support the rotor and cover. In testing, it was noticed that having a Savonius turbine mounted only at the bottom led to vibrations which resulted in a decrease in performance. By providing additional support to the rotor via an upper bearing, these vibrations are mitigated. Having the cover mounted to a bearing on the upper frame requires a much smaller bearing and more simple design compared to mounting on the lower frame.
Meet the Team Members
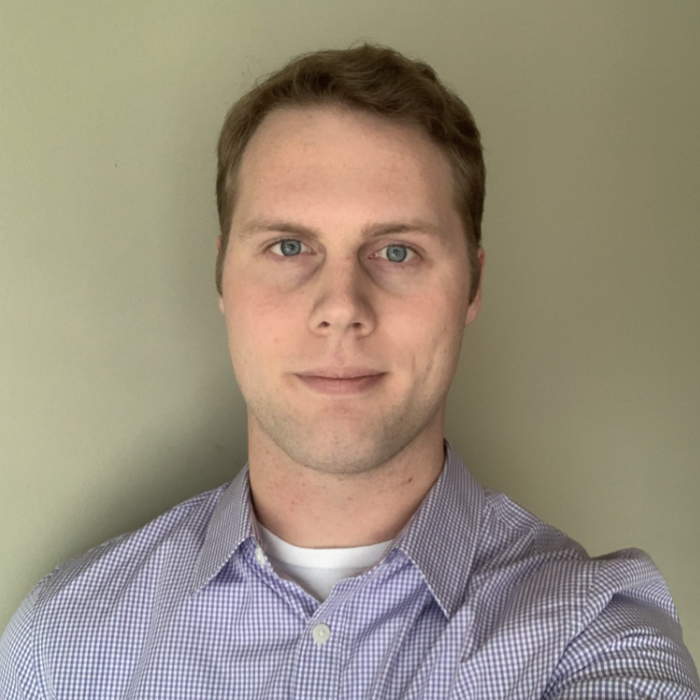


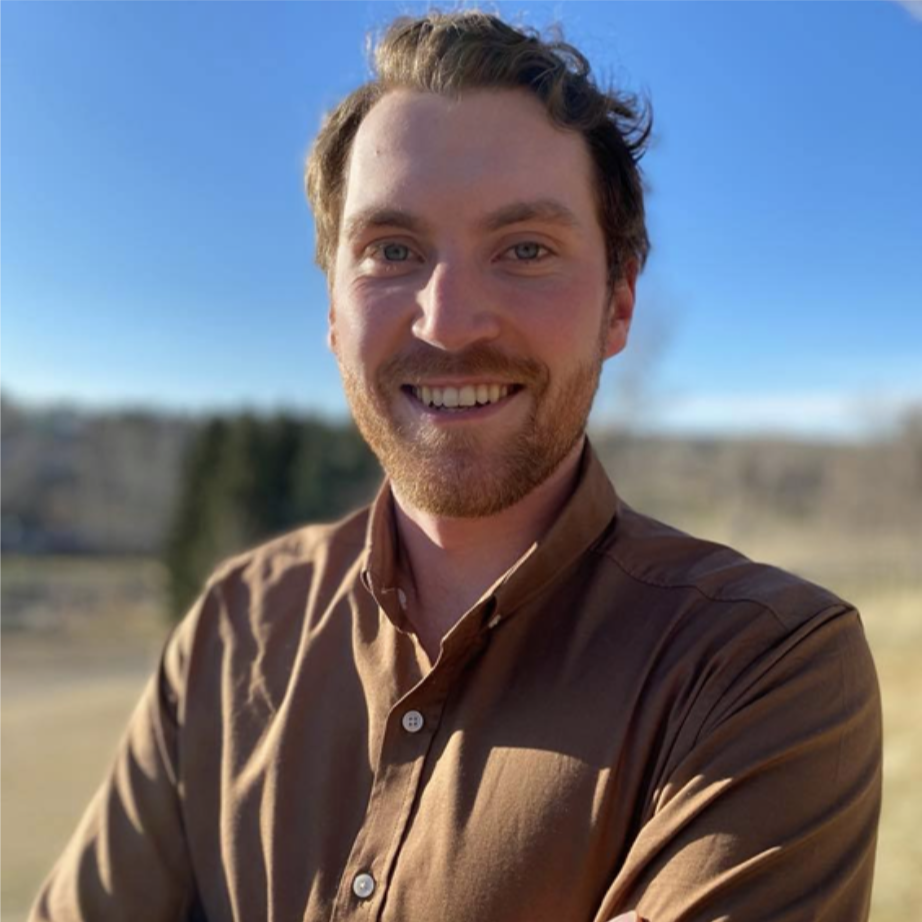
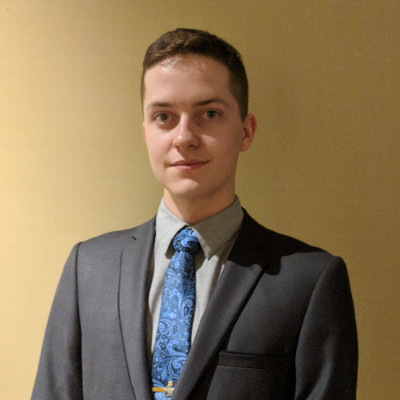
Partners and mentors
We would like to thank Dr. David Wood for providing essential advice and knowledge with his vast experience on Wind Turbines and renewable energy sources. We would also like to thank Itoje John and Temi Philips who helped us operate the wind tunnel and the Data Acquisition equipment. Finally, we would like to thank Dr. Simon Li and Danny Wong for their feedback throughout this project.