Project Category: Mechanical
Join Our Presentation
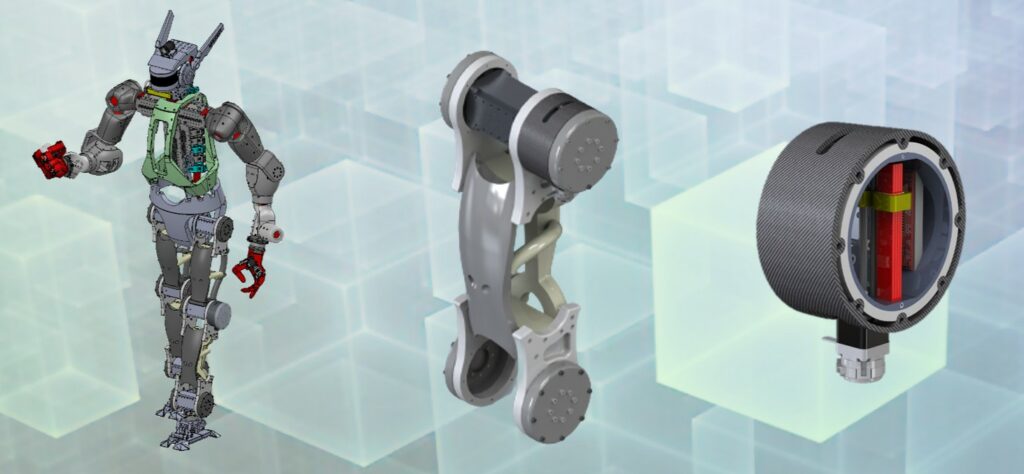
About Our Project
Compliance, which is the ability of a joint to move and flex under an eternal force without intentional movement by a muscle or motor, is what allows humans and complex robots move smoothly, interact completely with an unfamiliar environment, and effectively respond to unexpected external forces. The purpose of compliance is to allow joints to absorb external forces; the higher the compliance, the more force can be absorbed. Humans operate under varying degrees of compliance for various purposes, for example minimal compliance in the knee when running, and high compliance when walking on uneven surfaces. Compliance is the difference between our traditional perception of the rigid, shuffling, mechanical robot and those that will soon be able to outperform humans in terms of navigating challenging terrain.
The University of Calgary Robotarium lab has designed a full-scale humanoid robot for the purpose of pediatric care and inaccessible search-and-rescue. Our project goal is to create a device that can implement adjustable compliance in the robot knee, with the goal to be able to scale this device for all joints.
Our device connects the joint motor in the thigh to the robot’s shin through a very small cantilever (a beam attached at one end). A force on the shin will bend the beam, thus allowing for compliance. By including a device that can hold different parts of the beam still, the effective length of the beam is changed, creating stiffer compliance.
Meet Our Team Members
Scheduler and Project Accountant: Andrew has experience in the field of project management, assisting in cost estimates for multi-million-dollar projects at Plains Midstream Canada. It was his responsibility to monitor the projects deliverables to ensure they were met in a timely fashion. Andrew maintained the budget of the project by recording estimated/actual expenses and promoting financially-grounded decisions.
Engineering and Design Testing: Due to Alex’s high proficiency in engineering analysis, he was responsible for the calculations regarding material selection of the leaf spring, output of the motors, and encoding of the sliders position. Alex assisted in conductance of various tests on the leaf spring on the design itself. He also led the team in research, presentation, and selection of external components (motor, microcontroller, and motor driver)
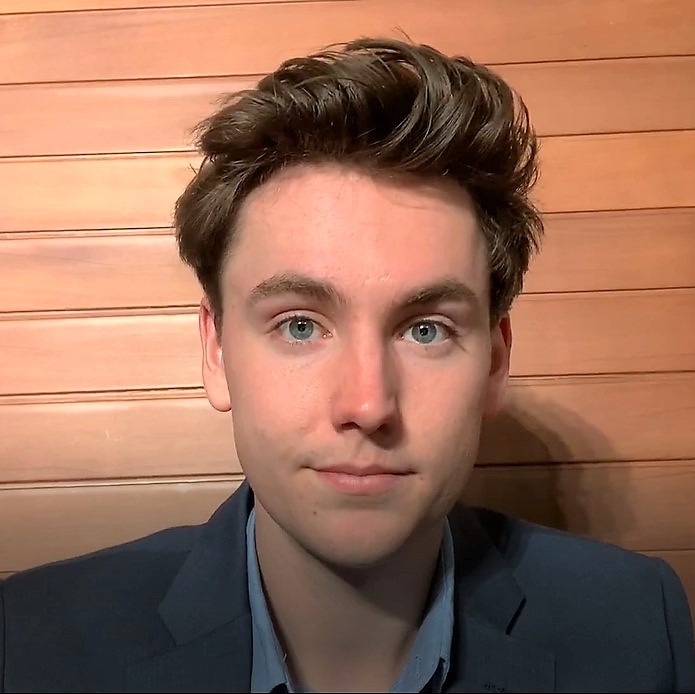
Procurement and Marketing: Along with contributing to the design process, Ty was responsible for the acquisitions of supplies and materials for theoretical design construction. Although a prototype was not required, Ty led the team in providing a detailed plan to the project sponsor for the construction of the device. Ty also led the organization and presentation of our final product and analytical results in the project website and video.
Research and Technical Writing: Although research was completed by each team member, Danielle was responsible for the compiling all background research documentation, as well as transcribing the research into technical writing that relates to the design (Section 2). In addition, Danielle assisted in conducting various simulations regarding the leaf spring deflection and material testing.
Initial Research and Communications: Kalindee was largely responsible for the research of human joint compliance values to compare our design to. Since the external research on the subject is limited, this includes analyzing videos and data that indicate ground force reactions of people in motion. Kalindee was also responsible for the communication to our sponsor, Dr. Ramirez-Serrano, by scheduling weekly meetings and sending e-mails on behalf of the team.
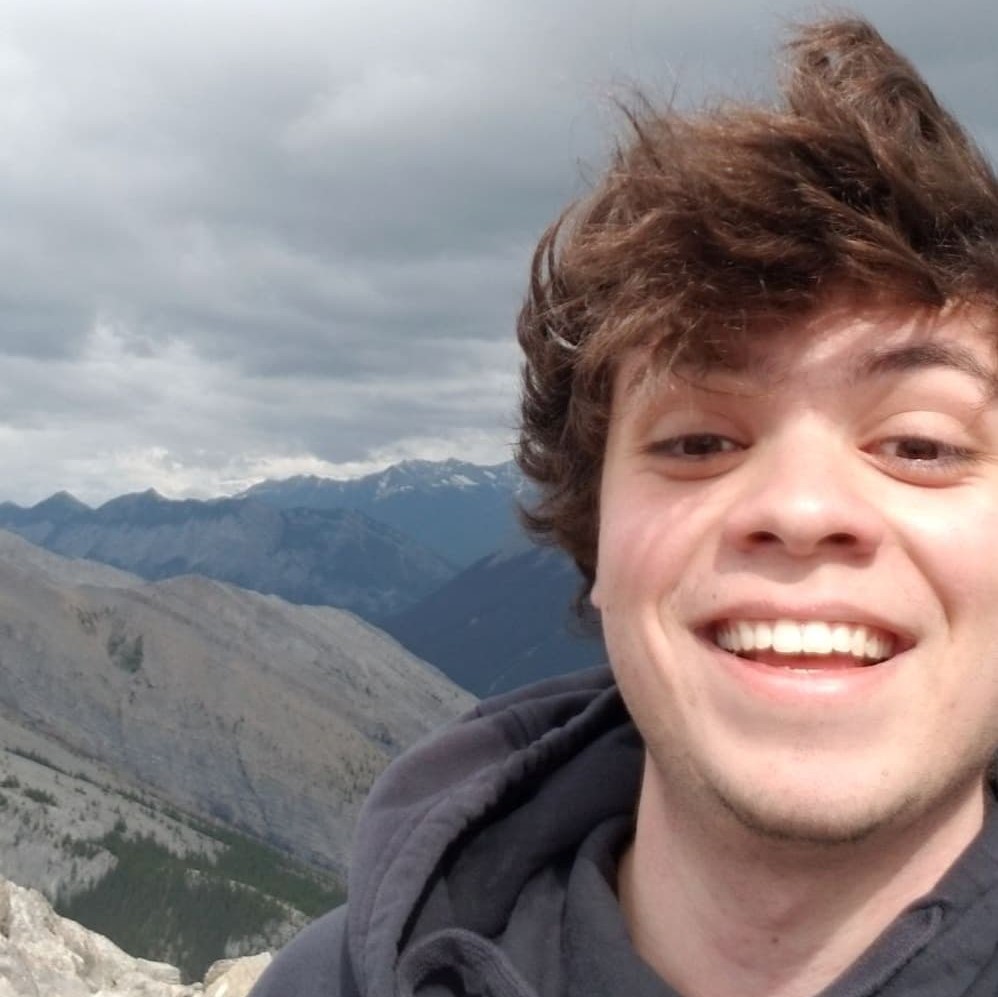
Lead Draftsman: Although the team has maintained a holistic approach to the design process, Brian was the main draftsman. Brian’s experience in CAD modelling through prior occupations and his involvement in engineering competition was a huge asset. Once team decisions were agreed upon, Brian was responsible for the modelling of concepts and revisions that remain frequent during the design process.
* click on a team member’s picture to be taken to their LinkedIn account for more information *
Details About Our Design
HOW OUR DESIGN ADDRESSES PRACTICAL ISSUES
The current design of the University of Calgary robot does not have compliance implemented in its joints. A small-scale version of the robot was tested in pediatric care, and reduced the time it took for children to be vaccinated by 62% (from 32 mins to 12 mins); however, in order to keep the children safe, it had to be powered off first, and many complained about it being lifeless or not being able to interact with it. Additionally, the goal of the Robotarium lab is to use the robot in search and rescue in locations inaccessible by humans. In the first three months of 2021 alone, the Kananaskis search-and-rescue team responded to 63 calls; however, it is often very difficult and expensive to accurately sense and maneuver rough terrain.
Our design to implement compliance in the robot solves these two issues. Not only will it allow for children to interact with the robot more realistically when it is powered off because the joints will flex under force (e.g. giving the robot a high five), but it will also make it safer to be left on, as the joints will bend and absorb force should they run into patients, doctors, or family. Additionally, compliance allows for a robot to maneuver rough terrain more easily and the robot does not have to perfectly sense and predict the ground, but rather can flex to unexpected changes in elevation. This is closer to how a human navigates uneven surfaces: getting a general idea through the sense, and flexing the ankles and knees to fit the ground as they step.
WHAT MAKES OUR DESIGN INNOVATIVE
Our design is innovative because it allows for adjustable compliance. Implementing compliance is easy, but creating a device that can change how stiff or loose a robotic joint is is much more challenging. It is important, however, because different situations call for different amount of compliance. For example, if the robot is running, the compliance in the knee must be very stiff (minimal compliance) otherwise the knee will buckle when the foot strikes the ground, whereas if the terrain is very uneven, the compliance in the knee must be very loose (large compliance).
Our device works by an internal motor moving a slider up and down the cantilevered beam, which can be controlled by external software. By moving this slider, the effective length of the beam is changed, meaning that the stiffness of this beam can change. Because the input motor (thigh) and external shaft (shin) are connected through this beam, the compliance can be altered easily. Additionally, the cantilever beam is designed to easily be replaced, meaning that if compliance needs to be drastically altered, a different material beam can be selected.
WHAT MAKES OUR DESIGN SOLUTION EFFECTIVE
Our design is effective for many reasons:
- It fits with the current motor and design of the current robot, with a small modification to the robot joint agreed upon by the Robotarium team.
- The total weight of the device is 335.44g (not including the chosen customized stepper motor to move the slider), meaning that every joint could contain a device like this with minimal impact on the robots overall weight.
- The device can be easily machined (confirmed by the U of C machine shop) with simple materials and common fasteners, bringing the total cost of the device to $202.49, under the project limit of $250 (excluding additional parts, like microcontroller, motor, and motor driver).
- It is a very small device, filling a total cylindrical volumetric space of approximately 440cm3 (radius = 47.25mm, width = 62.6mm). It is so small, in fact, that because it is designed to be placed within the robot’s pre-existing joint cavities, the joints are only extruded from the robot by 43.2mm, meaning that it does not affect the overall robot design.
- All aspects of the design meet the predetermined engineering specifications of strength, fatigue, failure, and compliance (see “Validation” and “Feasibility”).
HOW WE VALIDATED OUR DESIGN SOLUTION
Compliance
- One goal was to match human compliance ranges. Initial research was completed; however, very limited data is currently available, so we conducted a study on our own
- The maximum bound was found by analyzing Olympic runners at max speed. It was hypothesized that knee compliance would be near its minimum (stiffest), as a stiff knee joint is a sign of efficient form. Therefore, elite sprinters will have some of the lowest possible human knee compliance when running. By analyzing these sprinters in slow motion (to capture the angular deflection of their knee joints), corresponding force plate data, and known physical dimensions of the runners, the total moment around the runners knee was calculated done for a variety of runners and strides, and averaged to get a max human torsional spring constant in the knee of 1623 Nm/rad or 28.32 Nm/deg.
- The minimum bound was more difficult, as humans can technically have infinite compliance (0 N/m spring constant), so a practical situation that may demand a low spring constant in the robot’s knee must be analyzed to find the minimum spring constant it would conceivably need. This was found by analyzing a situation the robot could be in, where human compliance would likely be the highest: miscalculating their footing. The project sponsor hoped that our device would allow the robot would be able to walk up stairs normally, so by researching city residential and commercial buildings codes, it was determined that the max difference in possible step heights was 75mm. Assuming the robot sensed the step to be at the smallest possible height and it was actually at the highest, compliance needs to adjust for this miscalculation before significant weight has been shifted to the upper foot, otherwise the miscalculation will throw the robot off balance. The maximum amount of weight that can be shifted on an incorrect leg position (i.e. before the leg must be fully complied to the correct position to maintain balance) was approximated as less than or equal to 5% of the body weight, as found through testing our own human balance limits. Assuming an approximate limb position for the robot and knowing the total weight of the robot is 50kg, the minimum applicable human torsional spring constant in the knee was found (through testing all possible stair widths) to be 17.68Nm/rad or 0.3086Nm/deg.
Failure
- Another goal used to validate the design was a factor of safety of at least 1.2 for every part of the device
- Solidworks/Ansys simulations were run on over 20+ potential materials and beam geometries to determine cantilever deflection and stress throughout the cantilevered spring under a 135N load (the max possible applied force to the device, based on the robot joint motor’s max input torque of 7N).
- Material selection of the cantilever spring was based on its ability to meet this criteria (as well as provide an range accurate to human compliance).
- All test to find an acceptable material were performed on just the cantilever beam at the max effective length (68.93 mm) and input force (135N). Once Aluminum 7068-T6 was chosen, more rigorous Ansys testing with finer meshes, at all effective lengths and force locations, and including all connecting parts like the slider and outer shell was completed to ensure there was no stress concentrators or unexpected externalities that could cause failure.
FEASIBILITY OF OUR DESIGN SOLUTION
The design was measured to be feasible based on all the predetermined parameters:

Cost – The project came in $47.51 under budget, with a total cost of $202.49 for raw materials, manufacturing, and fasteners
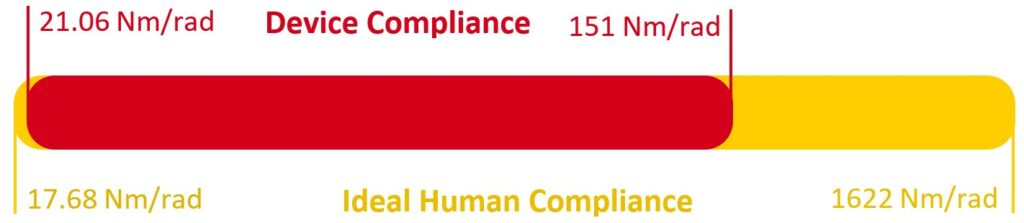
Compliance – The device can create compliance for a range of 21.06 Nm/rad to 151 Nm/rad, which is within desired range, and encompasses all practical applications. If human compliance extrema is desired the material of the cantilever can be changed to achieve this
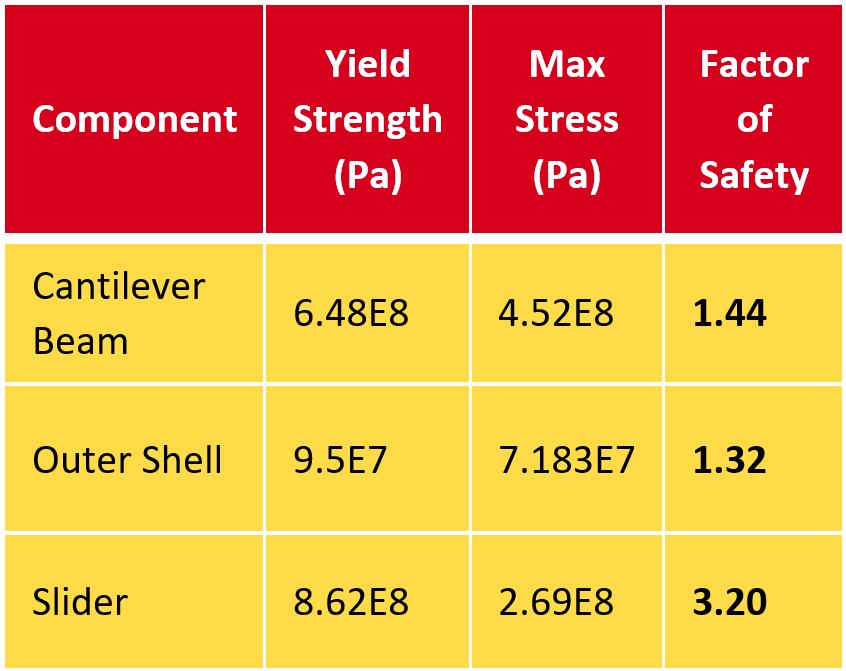
Failure – The parts experiencing maximum stress, the cantilever spring, the outer shell, and the slider, all have factors of safety greater than 1.2 (1.44, 1.32 and 3.20, respectively).
Partners and Mentors
We want to thank the many people who helped us with this project. Our project sponsor and head of the University of Calgary Robotarium Lab, Dr. Alex Ramirez-Serrano, not only provided valuable support and guidance, but also pushed us to innovate and explore beyond our comfort zones.
Our Photo Gallery
Full Design – Isometric View Full Design (Expanded) – Isometric Full Design (Attached to Robot) – Front Full Design (Attached to Robot) – Isometric Full Design (Attached to Robot) – Back Internal Design (Cap and Output Shaft Removed) – Front Internal Design (Cap and Output Shaft Removed) – Isometric Internal Design (Outer Shell Removed) – Isometric Internal Design (Extra Lighting) – Front Internal Design (Extra Lighting) – Isometric